General Information About Late Effects of Treatment for Childhood Cancer
During the past five decades, dramatic progress has been made in the development of curative therapies for pediatric malignancies. More than 80% of children with cancer who have access to contemporary therapies are expected to survive into adulthood.[1] The therapies responsible for this survival can also produce adverse, long-term, health-related outcomes, referred to as late effects, which appear months to years after completion of cancer treatment.
Many approaches have been used to study the very long-term morbidity associated with childhood cancer and its contribution to early mortality. These initiatives have used a spectrum of resources, including data from the following:
- Population-based registries.[2,3]
- Self-reported outcomes (provided through large-scale cohort studies).[4,5]
- Medical assessments.[6,7,8]
High-quality data is needed to establish the occurrence of and risk profiles for late cancer treatment–related toxicity. The highest quality data typically comes from studies that report outcomes in survivors who have undergone medical assessments that provide well-characterized clinical statuses, treatment exposures, and specific late effects. Regardless of study methodology, it is important to consider selection and participation bias of the cohort studies in the context of the findings.
Prevalence of Late Effects in Childhood Cancer Survivors
Late effects are common in adults who have survived childhood cancer. Their prevalence increases as time from cancer diagnosis elapses. Multi-institutional and population-based studies have shown excess risk of hospital-related morbidity among childhood and young adult cancer survivors compared with age- and sex-matched controls, with some evidence that this risk is disproportionately high among survivors of racial and ethnic minority populations.[3,9,10,11,12,13]
Among adults who were treated for cancer during childhood, late effects contribute to a high burden of morbidity. Research has shown the following:[4,7,14,15,16,17,18]
- 60% to more than 90% of survivors develop one or more chronic health conditions.
- 20% to 80% of survivors experience severe or life-threatening complications during adulthood.
- Morbidity accumulation is accelerated in young adult survivors of childhood cancer, compared with that of siblings and the general population. Accumulation of chronic diseases predicts risk of early mortality.[19]
The St. Jude Life (SJLIFE) cohort study aimed to describe the cumulative burden of cancer therapy using the cumulative burden metric, which incorporates multiple health conditions and recurrent events into a single metric that takes into account competing risks. By age 50 years, survivors in this cohort experienced an average of 17.1 chronic health conditions, 4.7 of which were severe/disabling, life threatening, or fatal.[16] This finding contrasts with the cumulative burden in matched community controls, who experienced 9.2 chronic health conditions, 2.3 of which were severe/disabling, life threatening, or fatal (see Figure 1).[16]
Figure 1. Figure shows distribution of cumulative burden by age among childhood cancer survivors of specific pediatric cancer subtypes and community controls participating in the SJLIFE cohort study. The cumulative burden at age 30 years and rate of cumulative burden growth is variable across cancer subtypes and organ systems. Reprinted from The Lancet, Volume 390, Issue 10112, Bhakta N, Liu Q, Ness KK, Baassiri M, Eissa H, Yeo F, Chemaitilly W, Ehrhardt MJ, Bass J, Bishop MW, Shelton K, Lu L, Huang S, Li Z, Caron E, Lanctot J, Howell C, Folse T, Joshi V, Green DM, Mulrooney DA, Armstrong GT, Krull KR, Brinkman TM, Khan RB, Srivastava DK, Hudson MM, Yasui Y, Robison LL, The cumulative burden of surviving childhood cancer: an initial report from the St Jude Lifetime Cohort Study (SJLIFE), Pages 2569–2582, Copyright (2017), with permission from Elsevier.
SJLIFE cohort study investigators compared the cumulative burden of chronic health conditions among 4,612 adolescent and young adult survivors at the ages of 18 years (the time of transition from pediatric to adult health care systems) and 26 years (the time of transition from family to individual health insurance plans) with that of 625 controls.[20]
- Survivors at the age of 18 years experienced an average of 22.3 disabling conditions per 100 individuals versus 3.5 in controls, and 128.7 lower-severity conditions (at risk of progressing to higher-grade disabling conditions) versus 12.4 in controls.
- Survivors at the age of 26 years experienced an average of 40.3 disabling conditions per 100 individuals versus 5.7 in controls, and 240.5 lower-severity conditions versus 51.3 in controls.
- The cumulative burden of disabling, disease-specific conditions at the ages of 18 and 26 years was most notable for survivors of bone tumors (musculoskeletal: 99.9 and 121.70, respectively), soft tissue sarcomas (musculoskeletal: 49.5 and 54.1, respectively), and central nervous system (CNS) tumors (neurological: 24.7 and 36.8, respectively).
- The cumulative burden of lower-severity conditions (potentially amenable to intervention) at the ages of 18 and 26 years was most notable for neurological conditions across most cancer subgroups, with the highest cumulative burden in CNS tumor survivors (95.2 and 162.3, respectively).
- These findings highlight the importance of optimizing access to health care and health insurance as survivors age and can no longer participate in pediatric health care systems.
The variability in prevalence is related to differences in the following:
- Age and follow-up time of the cohorts studied.
- Methods and consistency of assessment (e.g., self-reported vs. risk-based medical evaluations).
- Treatment intensity and treatment era.
Childhood Cancer Survivor Study
(CCSS) investigators demonstrated that the elevated risk of morbidity and mortality among aging survivors in the cohort increases beyond the fourth decade of life. By age 50 years, the cumulative incidence of a self-reported severe, disabling, life-threatening, or fatal health condition was 53.6% among survivors, compared with 19.8% among a sibling control group. Among survivors who reached age 35 years without a previous severe, disabling, life-threatening, or fatal health condition, 25.9% experienced a new grade 3 to grade 5 health condition within 10 years, compared with 6.0% of healthy siblings.[4]
The presence of serious, disabling, and life-threatening chronic health conditions adversely affects the health status of aging survivors. The greatest impact is on functional impairment and activity limitations. Predictably, chronic health conditions have been reported to contribute to a higher prevalence of emotional distress symptoms in adult survivors than in population controls.[21] Female survivors demonstrate a steeper trajectory of age-dependent decline in health status than do male survivors.[22] The even-higher prevalence of late effects among cohorts evaluated by clinical assessments is related to the subclinical and undiagnosed conditions detected by screening and surveillance measures.[7]
CCSS investigators also evaluated the impact of race and ethnicity on late outcomes. The study compared late mortality, subsequent neoplasms, and chronic health conditions in Hispanic (n = 750) and non-Hispanic Black (n = 694) participants with those in non-Hispanic White participants (n = 12,397).[23] The following results were observed:
- Cancer treatment did not account for disparities in mortality, chronic health conditions, or subsequent neoplasms observed among the groups.
- Differences in socioeconomic status and cardiovascular risk factors affected risk. All-cause mortality was higher among non-Hispanic Black participants than among other groups, but this difference disappeared after adjustment for socioeconomic status.
- Risk of developing diabetes was elevated among racial and ethnic minority groups even after adjustment for socioeconomic and obesity status.
- Non-Hispanic Black patients had a higher likelihood of reporting cardiac conditions, but this risk diminished after adjusting for cardiovascular risk factors.
- Nonmelanoma skin cancer was not reported by non-Hispanic Black participants, a finding that has been replicated by other studies.[24] Hispanic participants had a lower risk of nonmelanoma skin cancer than non-Hispanic White participants.
Recognition of late effects, concurrent with advances in cancer biology, radiological sciences, and supportive care, has resulted in a change in the prevalence and spectrum of treatment effects. In an effort to reduce and prevent late effects, contemporary therapy for most pediatric malignancies has evolved to a risk-adapted approach that is assigned on the basis of a variety of clinical, biological, and sometimes genetic factors.
The CCSS reported that with decreased cumulative dose and frequency of therapeutic radiation from 1970 to 1999, survivors have experienced a significant decrease in risk of subsequent neoplasms.[25]
- With the exception of survivors requiring intensive multimodality therapy for refractory/relapsed malignancies, life-threatening treatment effects are relatively uncommon after contemporary therapy in early follow-up (up to 10 years after diagnosis).
- However, survivors still frequently experience life-altering morbidity related to effects of cancer treatment on endocrine, reproductive, musculoskeletal, and neurological function.
A CCSS investigation examined temporal patterns in the cumulative incidence of severe to fatal chronic health conditions among survivors treated from 1970 to 1999.[26]
- The 20-year cumulative incidence of at least one grade 3 to 5 chronic condition decreased significantly, from 33.2% for survivors diagnosed between 1970 and 1979, to 29.3% for those diagnosed between 1980 and 1989, to 27.5% for those diagnosed between 1990 and 1999, compared with a 4.6% incidence in a sibling cohort.
- The overall decrease in incidence of chronic conditions across the three treatment decades was, in part, because of a substantial reduction of endocrinopathies, subsequent malignant neoplasms (SMNs), musculoskeletal conditions, and gastrointestinal conditions, whereas the cumulative incidence of hearing loss increased during this time.
- Declines in morbidity were not uniform across the diagnosis groups or condition types because of differences in treatment and survival patterns over time. For more information, see Figure 2.
- Despite declines in chronic health conditions over time, self-reported health status has not improved in more recent treatment eras. This finding may be because of the survival of children with higher-risk disease who would have previously died of cancer, or an enhanced awareness of and surveillance for late effects among more recently treated survivors.[27]
Figure 2. Cumulative incidence of grade 3–5 chronic health conditions in 5-year survivors of childhood cancer by diagnosis decade and siblings. (A) Cumulative incidence of a first grade 3–5 condition. (B) Cumulative incidence of two or more grade 3–5 conditions. The shaded area represents the 95% confidence interval (CI). The number of participants at risk (number censored) at each 5-year interval post-diagnosis is listed below the x-axis. The number censored does not include those who experienced a competing risk event (death from a cause other than a grade 5 chronic condition). Reprinted from The Lancet Oncology, Volume 19, Issue 12, Todd M Gibson, Sogol Mostoufi-Moab, Kayla L Stratton, Wendy M Leisenring, Dana Barnea, Eric J Chow, Sarah S Donaldson, Rebecca M Howell, Melissa M Hudson, Anita Mahajan, Paul C Nathan, Kirsten K Ness, Charles A Sklar, Emily S Tonorezos, Christopher B Weldon, Elizabeth M Wells, Yutaka Yasui, Gregory T Armstrong, Leslie L Robinson, Kevin C Oeffinger, Temporal patterns in the risk of chronic health conditions in survivors of childhood cancer diagnosed 1970–99: a report from the Childhood Cancer Survivor Study cohort. Pages 1590-1601, Copyright (2018), with permission from Elsevier.
Mortality
Late effects also contribute to an excess risk of premature death among long-term survivors of childhood cancer, as observed in the following studies:
- Several studies of large cohorts of survivors have reported early mortality among individuals treated for childhood cancer, compared with age- and sex-matched general population controls. Relapsed/refractory primary cancer remains the most frequent cause of death, followed by excess cause-specific mortality from subsequent primary cancers, and cardiac and pulmonary toxicity.[28,29,30,31,32,33]
- A CCSS study evaluated specific health-related causes of late mortality and excess deaths, compared with the general U.S. population to identify targets to reduce future risk.[33]
- At a median follow-up of 29 years from diagnosis, the 40-year cumulative all-cause mortality rate was 23.3%, with 51.2% of deaths attributed to health-related causes.
- Survivors who were 40 or more years from diagnosis experienced 131 excess health-related deaths per 10,000 person-years (95% confidence interval [CI], 111–163). Excess deaths were most commonly related to cancer (absolute excess risk per 10,000 person-years, 54; 95% CI, 41–68), heart disease (27; 18–38), and cerebrovascular disease (10; 5–17).
- Healthy lifestyle (assessed by smoking status, alcohol consumption, physical activity, body mass index, and absence of hypertension and diabetes) were each associated with a 20% to 30% reduction in health-related mortality, independent of other factors.
- An analysis of the CCSS and Surveillance, Epidemiology, and End Results (SEER) Program data evaluated conditional survival. The study demonstrated a subsequent 5-year survival rate of 92% or higher among most diagnoses at 5 years, 10 years, 15 years, and 20 years. Among those who had survived at least 5 years from diagnosis, the probability of all-cause mortality in the next 10 years was 8.8% in the CCSS and 10.6% in the SEER study, with neoplasms accounting for cause of death in approximately 75% of survivors.[34]
Despite high premature morbidity rates, overall mortality has decreased over time.[28,30,31,35,36]
CCSS investigators evaluated all-cause and health-related late mortality (including late effects of cancer therapy), SMNs, chronic health conditions, and neurocognitive outcomes among 6,148 survivors of childhood acute lymphoblastic leukemia (median age, 27.9 years; range, 5.9–61.9 years) diagnosed between 1970 and 1999.[37]
- Overall, the 20-year all-cause late mortality rate was 6.6%.
- Compared with participants who were treated in the 1970s, patients who were treated with risk-stratified regimens in the 1990s experienced lower health-related late mortality (rate ratio: 1990s standard risk, 0.2; 1990s high risk, 0.3), which was comparable to the U.S. population (standardized mortality ratio [SMR]: 1990s standard risk, 1.3; 1990s high risk, 1.7).
The risk of late mortality and serious chronic health conditions have decreased over time among survivors of acute myeloid leukemia (AML). CCSS investigators evaluated the long-term morbidity, mortality, and health status of more than 800 5-year survivors of childhood AML based on treatment and treatment era. Survivors were compared by treatment group (hematopoietic stem cell transplant [HSCT]); chemotherapy with cranial radiation [CRT]; chemotherapy only) and decade of diagnosis.[38]
- Among 856 survivors, the 20-year late mortality cumulative incidence was highest after HSCT (13.9%; chemotherapy with CRT, 7.6%; chemotherapy only, 5.1%).
- Mortality cumulative incidence decreased for survivors of HSCT diagnosed in the 1990s (8.5%) compared with the 1970s (38.9%), as did standardized mortality rates.
- Self-reported health status was good to excellent for 88.2% of childhood AML survivors, regardless of treatment.
- Most survivors did not experience any grade 3 to 5 chronic health conditions after 20 years (HSCT, 45.8%; chemotherapy with CRT, 23.7%; chemotherapy only, 27.0%).
- A temporal reduction in chronic health conditions cumulative incidence was seen after HSCT (1970s, 76.1%; 1990s, 38.3%; P = .02), mirroring a reduction in total-body irradiation use.
Population-based data from a state cancer registry was used to evaluate differences in survival and long-term outcomes by race and ethnicity among 4,222 children diagnosed with cancer between 1987 and 2012.[12]
- Compared with non-Hispanic White children, hospitalization was 70% (hazard ratio [HR], 1.7) more common 5 or more years after diagnosis for American Indian and Alaskan Native children and 50% (HR, 1.5) more common for Black children.
- Among survivors at 5 or more years from diagnosis, 2.3-fold to 3.6-fold statistically significant relative increases were observed for hospitalizations for specific conditions for American Indian and Alaskan Native children (HR, 2.3 for infection-related conditions; HR, 3.0 for hematologic-related conditions; HR, 2.6 for digestive-related conditions). The greatest increases were noted for mental health–related conditions (HR, 3.6), a pattern also noted for Black children (HR, 2.5).
An SJLIFE cohort study explored associations between modifiable chronic health conditions and late mortality within the context of social determinants of health.[39]
- Among 9,440 5-year childhood cancer survivors included in the analysis (median age at last follow-up, 27.5 years; median follow-up, 18.8 years), all-cause mortality (SMR, 7.6; 95% CI, 7.2–8.1) and health-related late mortality (SMR, 7.6; 95% CI, 7.0–8.2) was significantly higher than expected for U.S. mortality rates.
- Among 3,407 adult participants who completed an on-campus assessment (median age at assessment, 35.4 years; median follow-up, 27.3 years), significant increases in late all-cause and health-related deaths were associated with the number of modifiable chronic health conditions, living in a U.S. census block associated with a high area deprivation index, and frailty.
- Specific associations for excess health-related mortality included: one grade 2 modifiable chronic health condition (rate ratio [RR], 2.2; 95% CI, 1.1–4.4), two grade 2 modifiable chronic health conditions (RR, 2.5; 95% CI, 1.2–5.2), three grade 2 modifiable chronic health conditions (RR, 4.0; 95% CI, 1.9–8.4), area deprivation index in 51st to 80th percentile (RR, 9.2; 95% CI, 1.2–69.7), area deprivation index in 81st to 100th percentile (RR, 16.2; 95% CI, 2.1–123.7), and frailty (RR, 2.3; 95% CI, 1.2–4.1).
The CCSS and an SJLIFE cohort study investigated the contribution of cancer-predisposing variants to the risk of SMN-related late mortality (5 years or more after diagnosis).[40]
- Among 12,469 participants (6,172 male and 6,297 female), including 4,402 from the SJLIFE cohort (median follow-up time since collection of first biospecimen, 7.4 years) and 8,067 from the CCSS cohort (median follow-up time since collection of the first biospecimen, 12.6 years), 641 (5.1%) carried cancer-predisposing variants.
- Cancer-predisposing variants were significantly associated with an increased severity of SMNs (common terminology criteria for adverse events grade ≥4 vs. grade <4: odds ratio [OR], 2.15; 95% CI, 1.18–4.19).
- SMN-related deaths occurred in 263 participants (2.1%), and other-cause deaths occurred in 426 survivors (3.4%).
- At 10 years after the first biospecimen collection, the cumulative SMN-related mortality rate in carriers of cancer-predisposing variants was 3.7% (95% CI, 1.2%–8.5%) in SJLIFE and 6.9% (4.1%–10.7%) in CCSS. In comparison, the cumulative SMN-related mortality rate in noncarriers was 1.5% (1.0%–2.1%) in SJLIFE and 2.1% (1.7%–2.5%) in CCSS.
- Carrying a cancer-predisposing variant was associated with an increased risk of SMN-related mortality (SJLIFE: HR, 3.40; 95% CI, 1.37–8.43; CCSS: HR, 3.58; 95% CI, 2.27–5.63).
Survivors of adolescent and young adult (AYA) cancers
Little information is available on late mortality among survivors of AYA cancer.[41,42,43]
- Using SEER data, conditional relative survival up to 25 years after diagnosis was studied in a cohort of AYA patients (N = 205,954) diagnosed with a first malignant cancer (thyroid, melanoma, testicular, breast, lymphoma, leukemia, and CNS tumors).[41]
- For all cancer types combined, among individuals who survived up to 5 years, the subsequent 5-year relative survival rate exceeded 95% by 7 years after diagnosis.
- Most AYA cancer patients who survived at least 7 years after diagnosis experienced little difference in survival compared with the general population.
- For specific cancer types, including CNS tumors, female breast cancer, Hodgkin lymphoma, and leukemia, evidence of excess mortality risk persisted, or re-emerged, more than 10 years after a cancer diagnosis.
- Conditional relative survival was lowest for AYA patients with CNS tumors, although patients aged 15 to 29 years demonstrated a higher survival rate than did patients aged 30 to 39 years at the time of diagnosis of their CNS tumors.
- A separate analysis of 5-year survivors of AYA cancer (aged 15–39 years at diagnosis), also using SEER data (N = 282,969), demonstrated the following:[42]
- The 10-year all-cause mortality rate decreased from 8.3% for those diagnosed between 1975 and 1984 to 5.4% for those diagnosed between 2005 and 2011.
- The decrease in mortality primarily resulted from fewer deaths from the initial cancer.
- CCSS investigators compared chronic health conditions and all-cause and cause-specific mortality among 5,804 survivors of early-AYA cancer survivors (cancer diagnosis, age 15–20 years; median age, 42 years) and 5,804 childhood cancer survivors (cancer diagnosis, age <15 years; median age, 34 years) matched on primary cancer diagnosis.[43]
- The SMR was 5.9 (95% CI, 5.5–6.2) for early-AYA survivors and 6.2 (95% CI, 5.8–6.6) for younger childhood cancer survivors, compared with the general population.
- Early-AYA survivors had lower SMRs for death from health-related causes than did childhood cancer survivors (SMR, 4.8 [95% CI, 4.4–5.1] vs. 6.8 [95% CI, 6.2–7.4]), which was primarily evident more than 20 years after cancer diagnosis.
- Early-AYA and childhood cancer survivors were at greater risk of developing severe and disabling, life-threatening, or fatal (grades 3–5) health conditions than were siblings of the same age (HR, 4.2 [95% CI, 3.7–4.8] for early-AYA and 5.6 [95% CI, 4.9–6.3] for childhood cancer survivors), although the risk was lower for early-AYA survivors than for childhood cancer survivors.
- In a retrospective, population-based cohort study from Kaiser Permanente, cause-specific mortality in 2-year survivors (N = 10,574) of AYA cancers (patients aged 13–39 years who were diagnosed between 1990 and 2012) was examined and compared with individuals without cancer.[44]
- AYA cancer survivors were at a 10.4-fold increased risk of death compared with the matched noncancer cohort, and this risk remained elevated at more than 20 years after diagnosis (incidence rate ratio [IRR], 2.9).
- Beginning at 15 years after diagnosis, the incidence of second cancer–related mortality exceeded the rate of recurrence-related mortality.
- Mortality risk of suicide was doubled in AYA cancer survivors compared with the noncancer cohort.
- Chronic comorbidities were investigated in a retrospective, population-based cohort study of 6,778 2-year AYA cancer survivors diagnosed and monitored at Kaiser Permanente.[45]
- Approximately 17% of the survivors developed more than one comorbidity. The most common comorbidities were dyslipidemia (22 per 1,000 person-years), hypertension (16 per 1,000 person-years), diabetes (10 per 1,000 person-years), thyroid disorders (9 per 1,000 person-years), and severe depression or anxiety (8 per 1,000 person-years).
- IRRs were higher in survivors than in controls without a history of cancer for avascular necrosis (IRR, 8.25), followed by osteoporosis (IRR, 5.75), joint replacement (IRR, 3.89), stroke (IRR, 3.19), premature ovarian failure (IRR, 2.87), and cardiomyopathy or heart failure (IRR, 2.64).
- For survivors of AYA cancer, the prevalence of multiple comorbidities approached 40% at 10 years after index date (a 2-year time point from diagnosis), compared with 20% for those without cancer (P < .001).
Monitoring for Late Effects
Recognition of both acute and late modality–specific toxicity has motivated investigations evaluating the pathophysiology and prognostic factors for cancer treatment–related effects. Consequently, the results of late effects research have played an important role in the following areas:
- Changing pediatric cancer therapeutic approaches to reduce treatment-related mortality among survivors treated in more recent eras.[46]
- The development of risk counseling and health screening recommendations for long-term survivors by identifying the clinical and treatment characteristics of those at highest risk of therapy-related complications.[47]
The common late effects of pediatric cancer encompass several broad domains, including the following:
- Growth and development.
- Organ function.
- Reproductive capacity and health of offspring.
- Secondary carcinogenesis.
- Psychosocial sequelae related to the primary cancer, its treatment, or maladjustment associated with the cancer experience.
Late sequelae of therapy for childhood cancer can be anticipated based on therapeutic exposures, but the magnitude of risk and the manifestations in an individual patient are influenced by numerous factors. Multiple factors should be considered in the risk assessment for a given late effect (see Figure 3).[48]
Cancer-related factors:
- Organs or tissues affected by the cancer.
- Direct tissue effects.
- Cancer-induced organ dysfunction or other tissue effects.
Treatment-related factors:
- Radiation therapy: Total dose, fraction size, organ or tissue volume exposed.
- Chemotherapy: Agent type, dose-intensity, cumulative dose, schedule.
- Surgery: Technique, site, consequential organ dysfunction.
- HSCT.
- Combined-modality effects (therapeutic interactions).
- Blood product transfusion.
- Chronic graft-versus-host disease.
Host-related factors:
- Sex.
- Genetic predisposition.
- Premorbid, comorbid, posttreatment health states and exposures.
- Developmental status (age).
- Time from diagnosis/therapy.
- Inherent tissue sensitivities and capacity for normal tissue repair.
- Hormonal milieu.
- Socioeconomic status.
- Health habits.
Figure 3. Factors influencing morbidity and mortality of the childhood cancer survivor. Each arrow indicates a different factor affecting morbidity and mortality that exerts its effect along a continuum of care. Note that all effectors can begin exerting influence on morbidity during the period of cancer-directed therapy. Factors are separated into those that cannot be modified (red), those for which future interventions are plausible (yellow), and those for which there are known targets for interventions or areas in which therapy and surveillance have already been modified (blue). Reprinted from CA: A Cancer Journal for Clinicians, Volume 68, Issue 2, Dixon SB, Bjornard KL, Alberts NM, et al., Factors influencing risk-based care of the childhood cancer survivor in the 21st century, Pages 133–152, Copyright © 2018 American Cancer Society, with permission from John Wiley and Sons.
Resources to Support Survivor Care
Risk-based screening
The need for long-term follow-up of childhood cancer survivors is supported by the American Society of Pediatric Hematology/Oncology, the International Society of Pediatric Oncology, the American Academy of Pediatrics, the Children's Oncology Group (COG), and the Institute of Medicine. A risk-based medical follow-up is recommended, which includes a systematic plan for lifelong screening, surveillance, and prevention that incorporates risk estimates based on the following:[48]
- Previous cancer.
- Cancer therapy.
- Genetic predisposition.
- Lifestyle behaviors.
- Comorbid conditions.
- Sex.
Part of long-term follow-up also focuses on appropriate screening of educational and vocational progress. Specific treatments for childhood cancer, especially those that directly impact nervous system structures, may result in sensory, motor, and neurocognitive deficits that may have adverse effects on functional status, educational attainment, and future vocational opportunities. In support of this, a CCSS investigation observed the following:[49]
- Treatment with cranial radiation doses of 25 Gy or higher was associated with higher odds of unemployment (health related: OR, 3.47; 95% CI, 2.54–4.74; seeking work: OR, 1.77; 95% CI, 1.15–2.71).
- Unemployed survivors reported higher levels of poor physical functioning than employed survivors, had lower education and income, and were more likely to be publicly insured than unemployed siblings.
These data emphasize the importance of facilitating survivor access to individualized education services, which has been demonstrated to have a positive impact on education achievement.[50] These services may in turn enhance vocational opportunities.
In addition to risk-based screening for medical late effects, the impact of health behaviors on cancer-related health risks is also emphasized. Health-promoting behaviors are stressed for survivors of childhood cancer. Educational efforts focused on healthy lifestyle behaviors include the following:
- Abstinence from smoking, excess alcohol use, and illicit drug use to reduce the risk of organ toxicity and, potentially, subsequent neoplasms.
- Healthy dietary practices (e.g., a diet rich in plant foods and moderate in animal foods) [51] and active lifestyle to reduce treatment-related metabolic and cardiovascular complications.
- Regular physical activity to reduce neurocognitive problems and enhance psychological outcomes.[52,53]
- Survivors who engaged in consistent physical activity over time had fewer neurocognitive problems, including difficulties with task efficiency, emotional regulation, organization, and memory. They also experienced larger neurocognitive improvements, compared with those who had inconsistent activity levels.[52]
- Vigorous exercise in survivors has been associated with a lower prevalence of depression and somatization, as well as less impairment in physical functioning, general health and vitality, emotional role limitations, and mental health quality-of-life domains.[53]
Proactively addressing unhealthy and risky behaviors is pertinent because several research investigations confirm that long-term survivors use tobacco and alcohol and have inactive lifestyles despite their increased risk of cardiac, pulmonary, and metabolic late effects.[54,55,56]
Access to risk-based survivor care
Most childhood cancer survivors do not receive recommended risk-based care. The CCSS observed the following:
- 92.8% of survivors reported receiving some form of medical care in the previous year.[57]
- Nearly 40% reported receiving care that focused on their previous cancer (survivor-focused care).[57]
- Surveillance for new cases of cancer was very low in survivors at the highest risk of colon, breast, or skin cancer, suggesting that survivors and their physicians need education about the risk of subsequent neoplasms and recommended surveillance.[58]
- Sociodemographic factors have been linked to declining rates of follow-up care over time from diagnosis. CCSS participants who were male, had a household income of less than $20,000 per year, and had lower educational attainment (high school education or less) were more likely to report no care at their most recent follow-up survey. This trend is concerning because the prevalence of chronic health conditions increases with longer elapsed time from cancer diagnosis in adults treated for cancer during childhood.[59]
- A study that included 975 adult survivors of childhood cancer identified factors associated with attending the recommended risk-based, cancer-related medical visits. The relative risk of having a cancer-related visit was higher among survivors who:[60]
- Assigned a greater importance to these visits.
- Perceived a greater susceptibility to health problems.
- Had experienced a cancer-related chronic health problem that was moderate to life-threatening.
- Were seeing a primary care provider for a cancer-related problem.
- Had received a cancer treatment care plan.
- Expressed greater confidence in physicians' abilities to address questions and concerns.
Access to health insurance appears to play an important role in risk-based survivor care.[61,62] Lack of access to health insurance affects the following:
-
Cancer-related visits. In the CCSS, uninsured survivors were less likely than those privately insured to report a cancer-related visit (adjusted relative risk, 0.83; 95% CI, 0.75–0.91) or a cancer center visit (adjusted relative risk, 0.83; 95% CI, 0.71–0.98). Uninsured survivors had lower levels of utilization in all measures of care than privately insured survivors. In contrast, publicly insured survivors were more likely to report a cancer-related visit (adjusted relative risk, 1.22; 95% CI, 1.11–1.35) or a cancer center visit (adjusted relative risk, 1.41; 95% CI, 1.18–1.70) than were privately insured survivors.[61]
-
Health care outcomes. In a study comparing health care outcomes for long-term AYA cancer survivors with young adults who have no cancer history, the proportion of uninsured survivors did not differ between the two groups.[63]
-
Financial burden. Subgroups of adult survivors of childhood cancer may be at additional risk of health care barriers due to financial hardship. Younger survivors (aged 20–29 years), females, non-White survivors, and survivors reporting poorer health faced more cost barriers, which may inhibit the early detection of late effects.[63] Survivors are more likely than their siblings to forego needed medical care related to financial challenges.[64]
Overall, lack of health insurance—related to health issues, unemployment, and other societal factors—remains a significant concern for survivors of childhood cancer.[65,66] Legislation, including the Health Insurance Portability and Accountability Act (HIPAA),[67,68] has improved access and retention of health insurance among survivors, although the quality and limitations associated with these policies have not been well studied.
Transition to Survivor Care
Long-term follow-up programs
Transition of care from the pediatric to adult health care setting is necessary for most childhood cancer survivors in the United States.
When available, multidisciplinary long-term follow-up programs in the pediatric cancer center work collaboratively with community physicians to provide care for childhood cancer survivors. This type of shared care has been proposed as the optimal model to facilitate coordination between the cancer center oncology team and community physician groups providing survivor care.[69]
An essential service of long-term follow-up programs is the organization of an individualized survivorship care plan that includes the following:
- Details about therapeutic interventions undertaken for childhood cancer and their potential health risks (e.g., chemotherapy type and cumulative dose, radiation treatment fields and dose, surgical procedures, blood product transfusions, and HSCT).
- Personalized health screening recommendations.
- Information about lifestyle factors that modify risks.
A CCSS investigation that evaluated perceptions of future health and cancer risk highlighted the importance of continuing education of survivors during long-term follow-up evaluations. A substantial subgroup of adult survivors reported a lack of concern about future health (24%) and subsequent cancer risks (35%), even after exposure to treatments associated with increased risks. These findings present concerns that survivors may be less likely to engage in beneficial screenings and risk-reduction activities.[70]
The CCSS evaluated the surveillance and screening practices of 11,337 childhood cancer survivors. They found that fewer than half of high-risk survivors at increased risk of developing SMNs or cardiac dysfunction received the recommended surveillance, which likely exposes them to preventable morbidity and mortality.[58]
- 27% of survivors and 20% of primary care providers (PCP) had a survivorship care plan. Survivors treated after 1990 were more likely to have a survivorship care plan.
- Survivorship care plan possession by high-risk survivors was associated with increased adherence to COG-recommended breast (22% vs. 8%), skin (35% vs. 23%), and cardiac (67% vs. 33%) surveillance. PCP survivorship care plan possession was associated with increased adherence to skin surveillance (40% vs. 23%).
- Among high-risk survivors, adherence increased for colorectal (14% to 41%, P < .001) and cardiac (22% to 38%, P < .001) surveillance and decreased for breast surveillance (38% to 13%, P < .001) between 2007 and 2016.
- For average-risk survivors, better adherence to American Cancer Society recommendations for breast (57%), cervical (84%), and colorectal (69%) screening was observed than with COG recommendations. PCP survivorship care plan possession was associated with increased adherence to breast and colorectal screening. Survivors were less adherent to breast screening than the general population and less adherent to cervical screening than siblings.
For survivors who have not been provided with this information, the COG offers a template that can be used by survivors to organize a personal treatment summary. For more information, see the COG Survivorship Guidelines, Appendix 1.
COG long-term follow-up guidelines for childhood and AYA cancer survivors
To facilitate survivor and provider access to succinct information to guide risk-based care, COG investigators have organized a compendium of exposure- and risk-based health surveillance recommendations, with the goal of standardizing the care of childhood cancer survivors.[71]
The compendium of resources includes the following:
-
Long-Term Follow-Up Guidelines. COG Long-Term Follow-Up Guidelines for Survivors of Childhood, Adolescent, and Young Adult Cancers are appropriate for asymptomatic survivors presenting for routine exposure-based medical evaluation 2 or more years after completion of therapy.
-
Health Links. Patient education materials called Health Links provide detailed information on guideline-specific topics to enhance health maintenance and promotion among this population of cancer survivors.[72]
Information concerning late effects is summarized in tables throughout this summary.
Several groups have undertaken research to evaluate the yield from risk-based screening as recommended by the COG and other pediatric oncology cooperative groups.[7,73,74] Pertinent considerations in interpreting the results of these studies include the following:
- Variability in the cohort's age at treatment.
- Age at screening.
- Time from cancer treatment.
- Participation bias.
Collectively, these studies demonstrate that screening identifies a substantial proportion of individuals with previously unrecognized, treatment-related health complications of varying degrees of severity. Study results have also identified low-yield evaluations that have encouraged revisions of screening recommendations. Ongoing research is evaluating the cost effectiveness of screening in the context of consideration of benefits, risks, and harms.
References:
-
National Cancer Institute: NCCR*Explorer: An interactive website for NCCR cancer statistics. Bethesda, MD: National Cancer Institute. Available online. Last accessed December 15, 2023.
-
Lorenzi MF, Xie L, Rogers PC, et al.: Hospital-related morbidity among childhood cancer survivors in British Columbia, Canada: report of the childhood, adolescent, young adult cancer survivors (CAYACS) program. Int J Cancer 128 (7): 1624-31, 2011.
-
Rebholz CE, Reulen RC, Toogood AA, et al.: Health care use of long-term survivors of childhood cancer: the British Childhood Cancer Survivor Study. J Clin Oncol 29 (31): 4181-8, 2011.
-
Armstrong GT, Kawashima T, Leisenring W, et al.: Aging and risk of severe, disabling, life-threatening, and fatal events in the childhood cancer survivor study. J Clin Oncol 32 (12): 1218-27, 2014.
-
Teepen JC, Kok JL, Feijen EAM, et al.: Questionnaire- and linkage-based outcomes in Dutch childhood cancer survivors: Methodology of the DCCSS LATER study part 1. Cancer Med 12 (6): 7588-7602, 2023.
-
Geenen MM, Cardous-Ubbink MC, Kremer LC, et al.: Medical assessment of adverse health outcomes in long-term survivors of childhood cancer. JAMA 297 (24): 2705-15, 2007.
-
Hudson MM, Ness KK, Gurney JG, et al.: Clinical ascertainment of health outcomes among adults treated for childhood cancer. JAMA 309 (22): 2371-81, 2013.
-
Feijen EAM, Teepen JC, van Dulmen-den Broeder E, et al.: Clinical evaluation of late outcomes in Dutch childhood cancer survivors: Methodology of the DCCSS LATER 2 study. Pediatr Blood Cancer 70 (5): e30212, 2023.
-
Kurt BA, Nolan VG, Ness KK, et al.: Hospitalization rates among survivors of childhood cancer in the Childhood Cancer Survivor Study cohort. Pediatr Blood Cancer 59 (1): 126-32, 2012.
-
Zhang Y, Lorenzi MF, Goddard K, et al.: Late morbidity leading to hospitalization among 5-year survivors of young adult cancer: a report of the childhood, adolescent and young adult cancer survivors research program. Int J Cancer 134 (5): 1174-82, 2014.
-
Sørensen GV, Winther JF, de Fine Licht S, et al.: Long-Term Risk of Hospitalization Among Five-Year Survivors of Childhood Leukemia in the Nordic Countries. J Natl Cancer Inst 111 (9): 943-951, 2019.
-
Emerson MA, Olshan AF, Chow EJ, et al.: Hospitalization and Mortality Outcomes Among Childhood Cancer Survivors by Race, Ethnicity, and Time Since Diagnosis. JAMA Netw Open 5 (6): e2219122, 2022.
-
Streefkerk N, Tissing WJE, Korevaar JC, et al.: A detailed insight in the high risks of hospitalizations in long-term childhood cancer survivors-A Dutch LATER linkage study. PLoS One 15 (5): e0232708, 2020.
-
Berbis J, Michel G, Chastagner P, et al.: A French cohort of childhood leukemia survivors: impact of hematopoietic stem cell transplantation on health status and quality of life. Biol Blood Marrow Transplant 19 (7): 1065-72, 2013.
-
Phillips SM, Padgett LS, Leisenring WM, et al.: Survivors of childhood cancer in the United States: prevalence and burden of morbidity. Cancer Epidemiol Biomarkers Prev 24 (4): 653-63, 2015.
-
Bhakta N, Liu Q, Ness KK, et al.: The cumulative burden of surviving childhood cancer: an initial report from the St Jude Lifetime Cohort Study (SJLIFE). Lancet 390 (10112): 2569-2582, 2017.
-
Salloum R, Chen Y, Yasui Y, et al.: Late Morbidity and Mortality Among Medulloblastoma Survivors Diagnosed Across Three Decades: A Report From the Childhood Cancer Survivor Study. J Clin Oncol 37 (9): 731-740, 2019.
-
Streefkerk N, Teepen JC, Feijen EAM, et al.: The cumulative burden of self-reported, clinically relevant outcomes in long-term childhood cancer survivors and implications for survivorship care: A DCCSS LATER study. Cancer 130 (8): 1349-1358, 2024.
-
Esbenshade AJ, Lu L, Friedman DL, et al.: Accumulation of Chronic Disease Among Survivors of Childhood Cancer Predicts Early Mortality. J Clin Oncol 41 (20): 3629-3641, 2023.
-
Ehrhardt MJ, Williams AM, Liu Q, et al.: Cumulative burden of chronic health conditions among adolescent and young adult survivors of childhood cancer: Identification of vulnerable groups at key medical transitions. Pediatr Blood Cancer 68 (6): e29030, 2021.
-
Vuotto SC, Krull KR, Li C, et al.: Impact of chronic disease on emotional distress in adult survivors of childhood cancer: A report from the Childhood Cancer Survivor Study. Cancer 123 (3): 521-528, 2017.
-
Hudson MM, Oeffinger KC, Jones K, et al.: Age-dependent changes in health status in the Childhood Cancer Survivor cohort. J Clin Oncol 33 (5): 479-91, 2015.
-
Liu Q, Leisenring WM, Ness KK, et al.: Racial/Ethnic Differences in Adverse Outcomes Among Childhood Cancer Survivors: The Childhood Cancer Survivor Study. J Clin Oncol 34 (14): 1634-43, 2016.
-
Ehrhardt MJ, Bhakta N, Liu Q, et al.: Absence of Basal Cell Carcinoma in Irradiated Childhood Cancer Survivors of Black Race: A Report from the St. Jude Lifetime Cohort Study. Cancer Epidemiol Biomarkers Prev 25 (9): 1356-60, 2016.
-
Turcotte LM, Liu Q, Yasui Y, et al.: Temporal Trends in Treatment and Subsequent Neoplasm Risk Among 5-Year Survivors of Childhood Cancer, 1970-2015. JAMA 317 (8): 814-824, 2017.
-
Gibson TM, Mostoufi-Moab S, Stratton KL, et al.: Temporal patterns in the risk of chronic health conditions in survivors of childhood cancer diagnosed 1970-99: a report from the Childhood Cancer Survivor Study cohort. Lancet Oncol 19 (12): 1590-1601, 2018.
-
Ness KK, Hudson MM, Jones KE, et al.: Effect of Temporal Changes in Therapeutic Exposure on Self-reported Health Status in Childhood Cancer Survivors. Ann Intern Med 166 (2): 89-98, 2017.
-
Armstrong GT, Chen Y, Yasui Y, et al.: Reduction in Late Mortality among 5-Year Survivors of Childhood Cancer. N Engl J Med 374 (9): 833-42, 2016.
-
Holmqvist AS, Chen Y, Wu J, et al.: Late mortality after autologous blood or marrow transplantation in childhood: a Blood or Marrow Transplant Survivor Study-2 report. Blood 131 (24): 2720-2729, 2018.
-
Bagnasco F, Caruso S, Andreano A, et al.: Late mortality and causes of death among 5-year survivors of childhood cancer diagnosed in the period 1960-1999 and registered in the Italian Off-Therapy Registry. Eur J Cancer 110: 86-97, 2019.
-
Byrne J, Schmidtmann I, Rashid H, et al.: Impact of era of diagnosis on cause-specific late mortality among 77 423 five-year European survivors of childhood and adolescent cancer: The PanCareSurFup consortium. Int J Cancer 150 (3): 406-419, 2022.
-
Kilsdonk E, van Dulmen-den Broeder E, van Leeuwen FE, et al.: Late Mortality in Childhood Cancer Survivors according to Pediatric Cancer Diagnosis and Treatment Era in the Dutch LATER Cohort. Cancer Invest 40 (5): 413-424, 2022.
-
Dixon SB, Liu Q, Chow EJ, et al.: Specific causes of excess late mortality and association with modifiable risk factors among survivors of childhood cancer: a report from the Childhood Cancer Survivor Study cohort. Lancet 401 (10386): 1447-1457, 2023.
-
Mertens AC, Yong J, Dietz AC, et al.: Conditional survival in pediatric malignancies: analysis of data from the Childhood Cancer Survivor Study and the Surveillance, Epidemiology, and End Results Program. Cancer 121 (7): 1108-17, 2015.
-
Fidler MM, Reulen RC, Winter DL, et al.: Long term cause specific mortality among 34 489 five year survivors of childhood cancer in Great Britain: population based cohort study. BMJ 354: i4351, 2016.
-
Holmqvist AS, Chen Y, Wu J, et al.: Assessment of Late Mortality Risk After Allogeneic Blood or Marrow Transplantation Performed in Childhood. JAMA Oncol 4 (12): e182453, 2018.
-
Dixon SB, Chen Y, Yasui Y, et al.: Reduced Morbidity and Mortality in Survivors of Childhood Acute Lymphoblastic Leukemia: A Report From the Childhood Cancer Survivor Study. J Clin Oncol 38 (29): 3418-3429, 2020.
-
Turcotte LM, Whitton JA, Leisenring WM, et al.: Chronic conditions, late mortality, and health status after childhood AML: a Childhood Cancer Survivor Study report. Blood 141 (1): 90-101, 2023.
-
Ehrhardt MJ, Liu Q, Dixon SB, et al.: Association of Modifiable Health Conditions and Social Determinants of Health With Late Mortality in Survivors of Childhood Cancer. JAMA Netw Open 6 (2): e2255395, 2023.
-
Chen C, Qin N, Wang M, et al.: Cancer germline predisposing variants and late mortality from subsequent malignant neoplasms among long-term childhood cancer survivors: a report from the St Jude Lifetime Cohort and the Childhood Cancer Survivor Study. Lancet Oncol 24 (10): 1147-1156, 2023.
-
Anderson C, Smitherman AB, Nichols HB: Conditional relative survival among long-term survivors of adolescent and young adult cancers. Cancer 124 (14): 3037-3043, 2018.
-
Anderson C, Nichols HB: Trends in Late Mortality Among Adolescent and Young Adult Cancer Survivors. J Natl Cancer Inst 112 (10): 994-1002, 2020.
-
Suh E, Stratton KL, Leisenring WM, et al.: Late mortality and chronic health conditions in long-term survivors of early-adolescent and young adult cancers: a retrospective cohort analysis from the Childhood Cancer Survivor Study. Lancet Oncol 21 (3): 421-435, 2020.
-
Armenian SH, Xu L, Cannavale KL, et al.: Cause-specific mortality in survivors of adolescent and young adult cancer. Cancer 126 (10): 2305-2316, 2020.
-
Chao C, Bhatia S, Xu L, et al.: Chronic Comorbidities Among Survivors of Adolescent and Young Adult Cancer. J Clin Oncol 38 (27): 3161-3174, 2020.
-
Hudson MM, Armenian SH, Armstrong GT, et al.: Optimization of Health and Extension of Lifespan Through Childhood Cancer Survivorship Research. J Clin Oncol 36 (21): 2133-2134, 2018.
-
Kremer LC, Mulder RL, Oeffinger KC, et al.: A worldwide collaboration to harmonize guidelines for the long-term follow-up of childhood and young adult cancer survivors: a report from the International Late Effects of Childhood Cancer Guideline Harmonization Group. Pediatr Blood Cancer 60 (4): 543-9, 2013.
-
Dixon SB, Bjornard KL, Alberts NM, et al.: Factors influencing risk-based care of the childhood cancer survivor in the 21st century. CA Cancer J Clin 68 (2): 133-152, 2018.
-
Kirchhoff AC, Leisenring W, Krull KR, et al.: Unemployment among adult survivors of childhood cancer: a report from the childhood cancer survivor study. Med Care 48 (11): 1015-25, 2010.
-
Mitby PA, Robison LL, Whitton JA, et al.: Utilization of special education services and educational attainment among long-term survivors of childhood cancer: a report from the Childhood Cancer Survivor Study. Cancer 97 (4): 1115-26, 2003.
-
Lan T, Wang M, Ehrhardt MJ, et al.: Adherence to healthy diet and risk of cardiovascular disease in adult survivors of childhood cancer in the St. Jude Lifetime Cohort: a cross-sectional study. BMC Med 21 (1): 242, 2023.
-
Barlow-Krelina E, Chen Y, Yasui Y, et al.: Consistent Physical Activity and Future Neurocognitive Problems in Adult Survivors of Childhood Cancers: A Report From the Childhood Cancer Survivor Study. J Clin Oncol 38 (18): 2041-2052, 2020.
-
Tonorezos ES, Ford JS, Wang L, et al.: Impact of exercise on psychological burden in adult survivors of childhood cancer: A report from the Childhood Cancer Survivor Study. Cancer 125 (17): 3059-3067, 2019.
-
Lown EA, Hijiya N, Zhang N, et al.: Patterns and predictors of clustered risky health behaviors among adult survivors of childhood cancer: A report from the Childhood Cancer Survivor Study. Cancer 122 (17): 2747-56, 2016.
-
Gibson TM, Liu W, Armstrong GT, et al.: Longitudinal smoking patterns in survivors of childhood cancer: An update from the Childhood Cancer Survivor Study. Cancer 121 (22): 4035-43, 2015.
-
Devine KA, Mertens AC, Whitton JA, et al.: Factors associated with physical activity among adolescent and young adult survivors of early childhood cancer: A report from the childhood cancer survivor study (CCSS). Psychooncology 27 (2): 613-619, 2018.
-
Mueller EL, Park ER, Kirchhoff AC, et al.: Insurance, chronic health conditions, and utilization of primary and specialty outpatient services: a Childhood Cancer Survivor Study report. J Cancer Surviv 12 (5): 639-646, 2018.
-
Yan AP, Chen Y, Henderson TO, et al.: Adherence to Surveillance for Second Malignant Neoplasms and Cardiac Dysfunction in Childhood Cancer Survivors: A Childhood Cancer Survivor Study. J Clin Oncol 38 (15): 1711-1722, 2020.
-
Casillas J, Oeffinger KC, Hudson MM, et al.: Identifying Predictors of Longitudinal Decline in the Level of Medical Care Received by Adult Survivors of Childhood Cancer: A Report from the Childhood Cancer Survivor Study. Health Serv Res 50 (4): 1021-42, 2015.
-
Ford JS, Tonorezos ES, Mertens AC, et al.: Barriers and facilitators of risk-based health care for adult survivors of childhood cancer: A report from the Childhood Cancer Survivor Study. Cancer 126 (3): 619-627, 2020.
-
Casillas J, Castellino SM, Hudson MM, et al.: Impact of insurance type on survivor-focused and general preventive health care utilization in adult survivors of childhood cancer: the Childhood Cancer Survivor Study (CCSS). Cancer 117 (9): 1966-75, 2011.
-
Keegan TH, Tao L, DeRouen MC, et al.: Medical care in adolescents and young adult cancer survivors: what are the biggest access-related barriers? J Cancer Surviv 8 (2): 282-92, 2014.
-
Kirchhoff AC, Lyles CR, Fluchel M, et al.: Limitations in health care access and utilization among long-term survivors of adolescent and young adult cancer. Cancer 118 (23): 5964-72, 2012.
-
Nathan PC, Huang IC, Chen Y, et al.: Financial Hardship in Adult Survivors of Childhood Cancer in the Era After Implementation of the Affordable Care Act: A Report From the Childhood Cancer Survivor Study. J Clin Oncol 41 (5): 1000-1010, 2023.
-
Kirchhoff AC, Kuhlthau K, Pajolek H, et al.: Employer-sponsored health insurance coverage limitations: results from the Childhood Cancer Survivor Study. Support Care Cancer 21 (2): 377-83, 2013.
-
Kuhlthau KA, Nipp RD, Shui A, et al.: Health insurance coverage, care accessibility and affordability for adult survivors of childhood cancer: a cross-sectional study of a nationally representative database. J Cancer Surviv 10 (6): 964-971, 2016.
-
Park ER, Kirchhoff AC, Zallen JP, et al.: Childhood Cancer Survivor Study participants' perceptions and knowledge of health insurance coverage: implications for the Affordable Care Act. J Cancer Surviv 6 (3): 251-9, 2012.
-
Warner EL, Park ER, Stroup A, et al.: Childhood cancer survivors' familiarity with and opinions of the Patient Protection and Affordable Care Act. J Oncol Pract 9 (5): 246-50, 2013.
-
Jacobs LA, Shulman LN: Follow-up care of cancer survivors: challenges and solutions. Lancet Oncol 18 (1): e19-e29, 2017.
-
Gibson TM, Li C, Armstrong GT, et al.: Perceptions of future health and cancer risk in adult survivors of childhood cancer: A report from the Childhood Cancer Survivor Study. Cancer 124 (16): 3436-3444, 2018.
-
Landier W, Bhatia S, Eshelman DA, et al.: Development of risk-based guidelines for pediatric cancer survivors: the Children's Oncology Group Long-Term Follow-Up Guidelines from the Children's Oncology Group Late Effects Committee and Nursing Discipline. J Clin Oncol 22 (24): 4979-90, 2004.
-
Eshelman D, Landier W, Sweeney T, et al.: Facilitating care for childhood cancer survivors: integrating children's oncology group long-term follow-up guidelines and health links in clinical practice. J Pediatr Oncol Nurs 21 (5): 271-80, 2004 Sep-Oct.
-
Landier W, Armenian SH, Lee J, et al.: Yield of screening for long-term complications using the children's oncology group long-term follow-up guidelines. J Clin Oncol 30 (35): 4401-8, 2012.
-
Wasilewski-Masker K, Mertens AC, Patterson B, et al.: Severity of health conditions identified in a pediatric cancer survivor program. Pediatr Blood Cancer 54 (7): 976-82, 2010.
Subsequent Neoplasms
Subsequent neoplasms (SNs) are defined as histologically distinct neoplasms developing at least 2 months after completion of treatment for the primary malignancy. SNs may be benign or malignant. Childhood cancer survivors have increased risks of developing SNs that are multifactorial in etiology and vary according to the following:
- Host factors (e.g., genetics, immune function, hormone status).
- Primary cancer therapy.
- Environmental exposures.
- Lifestyle factors.
SNs are the leading cause of nonrelapse late mortality (standardized mortality ratio, 15.2; 95% confidence interval [CI], 13.9–16.6).[1] The Childhood Cancer Survivor Study (CCSS) reported the following 30-year cumulative incidence rates:[2]
- All SNs: 20.5% (95% CI, 19.1%–21.8%).
- Nonmelanoma skin cancer (NMSC): 9.1% (95% CI, 8.1%–10.1%).
- SNs with malignant histologies (excluding NMSC): 7.9% (95% CI, 7.2%–8.5%).
- Meningioma: 3.1% (95% CI, 2.5%–3.8%).
This represents a sixfold increased risk of SNs among cancer survivors, compared with the general population.[2]
Several studies have described the excess risk of SNs.[3,4]
Evidence (excess risk of SNs):
- A population-based study that leveraged registry data evaluated early second primary tumors occurring within 5 years of a first primary cancer diagnosed before age 15 years (1971–2010).[5]
- Early second primary tumors developed in 0.4% of cancer survivors, which is a sevenfold excess risk (standardized incidence ratio [SIR], 7.7; 95% CI, 6.7–8.9).
- Excess risk was higher among children diagnosed between 1981 and 1990 (SIR, 9.5; 95% CI, 7.1–12.5), compared with earlier or later decades (SIR, 6.5–7.5).
- Cancer predisposition syndromes were implicated in 21% of children with early second primary tumors and suspected in another 5%.
- An international case-control study pooled data for an analysis of meningioma risk after treatment for childhood cancer. The study evaluated the magnitude of radiation dose–response association, potential modifiers of radiation risks, and the role of chemotherapy.[6]
- Increasing radiation dose was associated with increased risk of meningioma (excess odds ratio [OR]/Gy, 1.44; 95% CI, 0.6–3.6), without evidence of departure from linearity.
- Exposure to radiation doses of 24 Gy or higher was associated with more than 30-fold higher odds of developing a meningioma (OR, 33.7; 95% CI, 14.1–80.3).
- Patients aged 10 years or older at treatment had a significantly lower radiation dose–response association compared with those treated before the age of 10 years (excess OR/Gy, 0.57; 95% CI, 0.18–1.91 vs. 2.20; 95% CI, 0.87–6.31).
- Treatment with methotrexate was associated with an increased risk of meningioma (OR, 3.43; 95% CI, 1.56–7.57), but without evidence of a dose-response association or interaction with radiation dose.
- Meningioma risk associated with radiation exposure remained significantly elevated 30 years after treatment (excess OR/Gy, 3.76; 95% CI, 0.77–29.15).
- A CCSS cohort reported on any new SN (including malignant neoplasms, NMSCs, benign meningiomas, and other benign neoplasms) occurring after age 40 years.[3]
- At the age of 55 years, the cumulative incidence of any new SN was 34.6%. The incidence of malignant SNs was 16.3%.
- Female sex and therapeutic radiation exposure were associated with an increased risk of developing SNs in multivariate analysis.
Prolonged follow-up has established that multiple SNs are common among aging childhood cancer survivors.[7,8]
- The CCSS also reported the following:[4]
- Individuals treated in more recent treatment eras experienced decreased risk of SNs (including subsequent malignancies, NMSCs, and benign meningiomas) compared with those treated earlier. This lower risk was attributed to decreased exposure to therapeutic radiation.
- However, individuals treated in the 1990s remain at increased risk of SNs compared with the general population.
- CCSS investigators evaluated morbidity and mortality associated with meningioma among 4,221 participants treated with cranial radiation therapy.[9]
- The cumulative incidence of subsequent meningioma by age 40 years was 5.6% in this group of patients, and the incidence was without demonstrable plateau.
- Risk factors for subsequent meningioma included female sex (hazard ratio [HR], 1.7; 95% CI, 1.2–2.3) and higher cranial radiation dose (HR, 2.6; 95% CI, 1.6–4.2 after 30 Gy or higher).
- Among survivors reporting meningiomas, the risk of neurological sequelae occurring 5 or more years after primary cancer diagnosis was increased for seizures (HR, 10.0; 95% CI, 7.0–15.3); auditory-vestibular-visual sensory deficits (HR, 2.3; 95% CI, 1.3–4.0); focal neurological dysfunction (HR, 4.9; 95% CI, 3.2–7.5); and severe headaches (HR, 3.2; 95% CI, 1.9–5.4).
- With a median follow-up of 72 months after meningioma diagnosis, 13% of the patients had died, with six deaths attributed to meningioma.
- Dutch Childhood Oncology Group (DCOG)–LATER investigators evaluated the cumulative meningioma incidence and performed excess relative risk modeling of benign meningiomas in childhood cancer patients.[10]
- Among 5,843 childhood cancer survivors (median follow-up, 23.3 years; range, 5.0–52.2 years), 97 patients developed a benign meningioma, including 80 after full-volume cranial radiation and 14 after partial-volume cranial radiation.
- The 40-year cumulative incidence of meningioma after any cranial radiation was 12.4% (95% CI, 9.8%–15.2%).
- Compared with cranial radiation doses of 1 to 19 Gy, no cranial radiation was associated with a low meningioma risk (HR, 0.04; 95% CI, 0.01–0.15), while increased risks were observed for doses of 20 to 39 Gy (HR, 1.66; 95% CI, 0.83–3.33) and 40 Gy or higher (HR, 2.81; 95% CI, 1.30–6.08).
- Survivors diagnosed before the age of 5 years showed significantly increased risks (HR, 2.38; 95% CI, 1.39–4.07) compared with patients diagnosed between the ages of 10 and 17 years.
- Radiation volume was not significantly associated with increased risk (HR for full vs. partial volume, 1.66; 95% CI, 0.86–3.22) and dose effects did not vary significantly according to exposure age or radiation volume.
- Treatment with carboplatin was associated with meningioma risk (HR, 3.55; 95% CI, 1.62–7.78), but a dose-response was not observed, and all nine exposed cases had received high-dose cranial radiation.
- In a follow-up study from the DCOG-LATER cohort, 90% of survivors with meningioma presented with symptoms rather than through late effects clinic screening, and 32% of survivors presented with synchronous meningiomas.[11]
- CCSS investigators have also evaluated associations between chemotherapy and subsequent malignant neoplasms (SMNs) among nonirradiated, long-term survivors.[12]
- Of 1,498 SMNs in 1,344 survivors, 229 occurred in 206 survivors who were treated with chemotherapy only.
- The 30-year SMN cumulative incidence was 3.9% for the chemotherapy-only group, 9.0% for the chemotherapy-plus-radiation group, 10.8% for the radiation-only group, and 3.4% for the neither-treatment group.
- SIRs for SMNs were increased for any SMN (SIR, 2.8), subsequent leukemia/lymphoma (SIR, 1.9), breast cancer (SIR, 4.6), soft tissue sarcoma (SIR, 3.4), thyroid cancer (SIR, 3.8), and melanoma (SIR, 2.3).
- The SMN rate was significantly associated with exposure to platinum chemotherapy doses higher than 750 mg/m2 (relative rate, 2.7) and alkylating agents (relative rate, 1.2 per 5,000 mg/m2).
- The breast cancer rate showed a linear dose response (relative rate, 1.3 per 100 mg/m2) with anthracycline exposure.
- DCOG-LATER investigators evaluated the contribution of chemotherapy to solid cancer risk in a large cohort of childhood cancer survivors diagnosed between 1963 and 2001 (median follow-up, 20.7 years).[13]
- The 25-year cumulative SN incidence was 3.9% and did not change across decades.
- Survivors treated with doxorubicin exhibited a dose-dependent increased risk of all solid cancers and breast cancer. This relationship was stronger in survivors with Li-Fraumeni syndrome–associated childhood cancers (leukemia, central nervous system [CNS], and sarcomas other than Ewing) than in survivors of other cancers.
- Among female survivors who did not receive chest radiation or total-body irradiation (TBI) and developed breast cancer (n = 31), HRs for doxorubicin dose tertiles were 1.3 (95% CI, 0.3–6.1), 5.6 (95% CI, 1.9–16.2), and 9.9 (95% CI, 4.2–23.8).
- A dose-response relationship was confirmed with cyclophosphamide and subsequent sarcoma, particularly bone sarcoma. The HR for subsequent sarcoma was 3.1 (95% CI, 1.5–6.0) for survivors who received cyclophosphamide at a dose greater than 9,400 mg/m2 and 2.6 (95% CI, 1.3–5.2) for those who received ifosfamide.
- St. Jude Life (SJLIFE) cohort study investigators assessed the contribution of pathogenic and likely pathogenic variants in cancer predisposition genes to SN risk in childhood cancer survivors.[14]
- Of 3,006 study participants evaluated by whole-genome sequencing (30-fold), 1,120 SNs were diagnosed among 439 survivors (14.6%), and 175 pathogenic or likely pathogenic variants were identified in 5.8% of survivors. The prevalence of a pathogenic or likely pathogenic variant among nonirradiated survivors with SNs was much higher, at 18%.
- Variants were associated with significantly increased rates of breast cancer (relative risk [RR], 13.9) and sarcoma (RR, 10.6) among irradiated survivors and with developing any SN (RR, 4.7), breast cancer (RR, 7.7), nonmelanoma skin cancer (RR, 11), and two or more histologically distinct SNs (RR, 18.6).
- Variant carriers did not have an increased rate of meningioma or thyroid cancer.
The incidence and type of SNs depend on the following:
- Primary cancer diagnosis.
- Type of therapy received.
- Presence of genetic conditions.
Unique associations with specific therapeutic exposures have resulted in the classification of SNs into the following two distinct groups:
- Myelodysplastic syndrome postcytotoxic therapy (MDS-pCT) and acute myeloid leukemia postcytotoxic therapy (AML-pCT) (previously termed t-MDS and t-AML).
- Therapy-related solid SNs.
Myelodysplastic Syndrome and Acute Myeloid Leukemia Postcytotoxic Therapy (MDS-pCT and AML-pCT)
Subsequent primary leukemias have been reported in survivors of Hodgkin lymphoma, leukemia, sarcoma, CNS tumors, non-Hodgkin lymphoma, neuroblastoma, and Wilms tumor. In a cohort of nearly 70,000 5-year childhood cancer survivors, survivors had a fourfold increased risk (SIR, 3.7) of developing a leukemia, with an absolute excess risk of 7.5. Specifically, a sixfold relative risk of developing a myeloid leukemia (SIR, 5.8) was reported.[15]
- The risk remained significantly elevated beyond 20 years from the first primary malignancy (SIR, 2.4).
- Hodgkin lymphoma survivors had the greatest excess risk of developing a subsequent leukemia (SIR, 7.9) and specifically a myeloid leukemia (SIR, 12.1), with the largest excess risk seen before 20 years of follow-up.
A pooled analysis examined all published studies with detailed treatment data for children with cancer diagnosed between 1930 and 2000. Treatment data included estimated radiation doses to the active bone marrow and doses of specific chemotherapy agents. In this report, 147 cases of second primary leukemia (69% of cases were AML) were matched to 522 controls.[16]
- After controlling for other therapies, topoisomerase II inhibitor therapy was associated with an increased risk of second AML (no radiation and >2,500 mg/m2 vs. none: OR, 14.3; 95% CI, 2.7–75.1).
- Radiation dose to the active bone marrow was also associated with an increased risk of second AML among patients who did not receive chemotherapy (>0–12 Gy: OR, 4.9; 95% CI, 0.95–25.6) but not among those who received chemotherapy.
- Second primary leukemias were most likely to occur in the first decade after cancer treatment.
Characteristics of MDS-pCT and AML-pCT include the following:[17,18]
- A short latency (<10 years from primary cancer diagnosis). The risk of MDS-pCT or AML-pCT plateaus after 10 to 15 years. Although the risk of subsequent leukemia remains significantly elevated beyond 15 years from primary diagnosis (SIR, 3.5; 95% CI, 1.9–6.0), these events are relatively rare, with an absolute excess risk of 0.02 cases per 1,000 person-years.[18]
- An association with alkylating agents and/or topoisomerase II inhibitors.
Based on the updated definitions from the World Health Organization, MDS-pCT and AML-pCT are clonal disorders, which arise in patients previously exposed to cytotoxic therapy, either chemotherapy or large-field radiation therapy, for an unrelated neoplasm.[19] The following two types of MDS-pCT and AML-pCT are the most frequently observed among survivors:
-
Alkylating agent–related type: Alkylating agents associated with MDS-pCT and AML-pCT include cyclophosphamide, ifosfamide, mechlorethamine, melphalan, busulfan, nitrosoureas, chlorambucil, and dacarbazine.[20]
The risk of alkylating agent–related MDS or AML is dose dependent, with a latency of 3 to 5 years after exposure; it is associated with abnormalities involving chromosomes 5 (-5/del(5q)) and 7 (-7/del(7q)).[20]
-
Topoisomerase II inhibitor–related type: Topoisomerase II inhibitor agents include etoposide, teniposide, and anthracycline-related drugs.
Most of the translocations observed in patients exposed to topoisomerase II inhibitors disrupt a breakpoint cluster region between exons 5 and 11 of the band 11q23 and fuse KMT2A with a partner gene.[20] Topoisomerase II inhibitor–related AML presents as overt leukemia after a latency of 6 months to 3 years and is associated with balanced translocations involving chromosome bands 11q23 or 21q22.[21]
For more information, see the Therapy-Related AML and Therapy-Related Myelodysplastic Neoplasms section in Childhood Acute Myeloid Leukemia Treatment.
Therapy-Related Solid SNs
Therapy-related solid SNs represent 80% of all SNs, demonstrate a strong relationship with radiation exposure, and are characterized by a latency that exceeds 10 years. The risk of solid SNs continues to increase with longer follow-up. The risk of solid SNs is highest when the following occur:[4,13]
- Younger age at time of radiation exposure.
- High total dose of radiation.
- Longer period of follow-up after radiation exposure.
The histological subtypes of solid SNs encompass a neoplastic spectrum ranging from benign and low-grade malignant lesions (e.g., NMSC, meningiomas) to high-grade malignancies (e.g., breast cancers, glioblastomas) (see Figure 4).[4,13,22,23]
Figure 4. Fitted radiation dose-response by type of second cancer, based on previously published studies of second sarcoma, skin, meningioma, salivary gland, glioma, breast, and thyroid gland. The order of second cancers from top to bottom in the graph is the same as in the key to the right of the panel. Reprinted from International Journal of Radiation Oncology*Biology*Physics, Volume 94, Issue 4, Inskip PD, Sigurdson AJ, Veiga L, et al., Radiation-Related New Primary Solid Cancers in the Childhood Cancer Survivor Study: Comparative Radiation Dose Response and Modification of Treatment Effects, Pages 800–807, Copyright © 2016, with permission from Elsevier.
Solid SNs in childhood cancer survivors most commonly involve the following:[4,13,22,24,25,26,27]
- Breast.
- Thyroid.
- CNS.
- Bone and soft tissue.
- Skin.
With longer follow-up of adult survivors of childhood cancer cohorts, epithelial neoplasms have been observed in the following:[13,28]
- Lung.
- Gastrointestinal tract.
- Oral cavity.
- Genitourinary system.
- Kidney.
Benign and low-grade SNs, including NMSCs and meningiomas, have also been observed with increasing prevalence in survivors who were treated with radiation therapy for childhood cancer.[4,10,29]
Subsequent neoplasms after hematopoietic stem cell transplant (HSCT)
Recipients of HSCT are treated with high-dose chemotherapy and, often, TBI, which makes their risk of SNs unique from that of the general oncology population.
- Among 4,318 first-time allogeneic HSCT recipients treated for AML and chronic myeloid leukemia between 1986 and 2005, and conditioned with high-dose busulfan and cyclophosphamide (Bu-Cy), 66 solid cancers were reported at a median of 6 years post-HSCT.[30]
- The cumulative incidence of new solid cancers was 0.9% at 5 years and 2.4% at 10 years and appears to be similar, regardless of exposure to radiation.
- Bu-Cy conditioning without TBI was associated with higher risks of solid SNs than in the general population.
- Chronic graft-versus-host disease increased the risk of SNs, especially those involving the oral cavity.
- A study of 4,905 1-year survivors of allogeneic HSCT who underwent transplant between 1969 and 2014 for malignant or nonmalignant diseases, and were followed for a median 12.5 years, demonstrated a strong effect of TBI dose and dose fractionation on risk of SNs.[31]
- The 20-year cumulative incidence of SN after HSCT for individuals treated at younger than 20 years was 8.1%.
- SN risk was highest in survivors exposed to high-dose, single-fraction TBI (6–12 Gy) or very high-dose fractionated TBI (14.4–17.5 Gy).
- With low-dose TBI (2–4.5 Gy), the SN risk was comparable to the risk with chemotherapy alone, although it was still twofold higher than in the general population.
- Among individuals treated at younger than 20 years, the number of SNs was 12.5-fold higher than expected in the general population, and the excess absolute risk was 10.6 per 1,000 person-years. Survivors treated with HSCT at this young age were more likely to develop SNs than were survivors who were treated after age 50 years (HR, 2.3).
Some well-established solid SNs are described in the following sections.
Breast cancer
Female survivors of childhood, adolescent, and young adult cancer treated with radiation therapy to fields including the chest are at increased risk of developing breast cancer.
- The cumulative breast cancer incidence ranges from 8% to 20% by age 40 to 45 years among childhood cancer survivors and is as high as 35% by age 50 years in Hodgkin lymphoma survivors, comparable to that observed among BRCA gene variant carriers.[17,32,33,34,35]
- Radiation dose and volume of breast exposed are important factors affecting risk. Specific chemotherapeutic agents, particularly alkylating agents and anthracyclines, may affect risk as well.[32,34,36]
Evidence (excess risk of breast cancer):
- Breast cancer is the most common therapy-related solid SN after a previous diagnosis of Hodgkin lymphoma.[17,33] The following has been observed in female survivors of childhood Hodgkin lymphoma:
- Excess risk of breast cancer has been reported in survivors treated with high-dose, extended-volume radiation at age 30 years or younger.[37]
- Data indicate that females treated with low-dose, involved-field radiation also exhibit excess breast cancer risk.[38]
- Patients who received limited volume supradiaphragmatic radiation therapy (excluding the axillae) had a significantly lower risk of subsequent breast cancers than patients who received full mantle-field radiation therapy.[36]
- For patients treated with radiation therapy to the chest before age 16 years, the cumulative incidence of breast cancer approaches 20% by age 45 years.[17]
- The latency period after chest irradiation ranges from 8 to 10 years, and the risk of subsequent breast cancer increases in a linear fashion with radiation dose (P for trend < .001).[39]
- Treatment with higher cumulative doses of alkylating agents and ovarian radiation of 5 Gy or higher (exposures predisposing to premature menopause) have been correlated with reductions in breast cancer risk, underscoring the potential contribution of hormonal stimulation on breast carcinogenesis.[36,40,41]
- The observed number of invasive breast cancer cases among young (aged <30 years) survivors, compared with what is expected in the general population, has decreased over recent treatment eras (1970s SIR, 55.0 vs. 1990s SIR, 14.3).[35]
- The risk of breast cancer was also increased in the following studies that used lower radiation doses to treat cancer that metastasized to the chest/lung (e.g., Wilms tumor, sarcoma) and exposed the breast tissue:
- In 116 children in the CCSS cohort treated with 2 Gy to 20 Gy to the lungs (median, 14 Gy), the SIR for breast cancer was 43.6 (95% CI, 27.1–70.1).[34]
- A report of 2,492 female participants in the National Wilms Tumor Studies 1 through 4 (1969–1995) addressed the excess risk of breast cancer.[42]
- Sixteen of 369 women who received chest irradiation for metastatic Wilms tumor developed invasive breast cancer (cumulative risk at age 40 years, 14.8% [95% CI, 8.7%–24.5%]). The SIR of 27.6 (95% CI, 16.1–44.2) was based on 5,010 person-years of follow-up.
- Of the 369 patients, radiation doses to the chest were less than 12 Gy in 4%, 12 Gy in 64%, 13 Gy to 15 Gy in 19%, and more than 15 Gy in 13% of patients.
- For all patients who developed breast cancer (with or without chest irradiation), the median age at first breast cancer diagnosis was 34.3 years (range, 15.5–48.4) and the median time from Wilms tumor diagnosis was 27.1 years (range, 7.9–35.7).
- An international collaborative study pooled individual patient data from 17,903 childhood cancer survivors. The study evaluated the dose-dependent effects of individual anthracycline agents on the development of subsequent breast cancer and interactions with chest radiation therapy. There were 782 survivors (4.4%) who developed a subsequent breast cancer.[43]
- Doxorubicin was associated with a dose-dependent increase of subsequent breast cancer risk (HR per 100 mg/m2, 1.24; 95% CI, 1.18–1.31).
- There was a more-than-twofold increased risk for survivors treated with 200 mg/m2 or higher cumulative doxorubicin dose, compared with no doxorubicin (HR, 2.50 for 200–299 mg/m2; HR, 2.33 for 300–399 mg/m2; HR, 2.78 for ≥400 mg/m2).
- The associations were not statistically significant for daunorubicin, whereas epirubicin was associated with increased subsequent breast cancer risk (exposure yes vs. no: HR, 3.25; 95% CI, 1.59–6.63).
- The HRs per 100 mg/m2 of doxorubicin were 1.11 (95% CI, 1.02–1.21) for patients treated with chest radiation therapy and 1.26 (95% CI, 1.17–1.36) for patients who did not receive chest radiation therapy.
- The risk of developing breast cancer after radiation therapy and chemotherapy with anthracyclines was evaluated in the CCSS. In a nested-case control study of 271 childhood cancer survivors (diagnosed between 1970–1986) who were subsequently diagnosed with breast cancer, the combination of anthracyclines and radiation therapy to the breast was associated with increased risks of breast cancer consistent with an additive interaction.[32]
- For the study group, the median age of first cancer diagnosis was 15 years and the median age at breast cancer diagnosis was 39 years.
- The OR for breast cancer increased with increasing radiation dose to the breast (OR per 10 Gy, 3.9; 95% CI, 2.5–6.5) and was similar for estrogen receptor–positive and estrogen receptor–negative cancers.
- The OR per 10 Gy to the breast was higher for women who received ovarian doses less than 1 Gy (OR, 6.8; 95% CI, 3.9–12.5) than for women who received ovarian doses greater than or equal to 15 Gy (OR, 1.4; 95% CI, 1.0–6.4).
- The OR for breast cancer increased with cumulative anthracycline dose (OR per 100 mg/m2, 1.23; 95% CI, 1.09–1.39; P < .01 for trend).
- There was an additive interaction between radiation therapy and anthracycline treatment. The OR was 19.1 (95% CI, 7.6–48.0) for the combined association of anthracycline therapy and breast radiation dose of 10 Gy or more (compared with 0 to less than 1 Gy) versus 9.6 (95% CI, 4.4–20.7) without anthracycline therapy.
- Childhood cancer survivors not exposed to chest radiation also have an increased risk of breast cancer at a young age.
- The SJLIFE study assessed subsequent breast cancer risk among 1,467 female cancer survivors and evaluated risk associated with anthracycline exposure. The study also evaluated whether surveillance imaging affects breast cancer outcomes.[24]
- In women who received neither chest radiation nor anthracyclines, the cumulative incidence of breast cancer was 2% at age 35 years and 15% at age 50 years. For women who were treated with 250 mg/m2 or higher of anthracyclines, the rates were 7% at age 35 years and 46% at age 50 years.
- Anthracycline doses of 250 mg/m2 or higher remained significantly associated with increased risk of breast cancer in models, excluding survivors with cancer predisposition gene variants, chest radiation of 10 Gy or higher, or both.
- Breast cancers detected by imaging and/or prophylactic mastectomy were more likely to be in situ carcinomas, be smaller masses, have no lymph node involvement, and be treated without chemotherapy, compared with breast cancers detected by physical findings.
- Dual imaging with mammography and breast magnetic resonance imaging (MRI) in this cohort was a sensitive and specific approach to identify breast cancers that require less aggressive therapy than breast cancers detected by physical findings.
- A CCSS investigation examined the breast cancer risk of 3,768 female participants who did not receive chest radiation.[44]
- A fourfold excess risk (SIR, 4.0; 95% CI, 3.0–5.3) of breast cancer was observed compared with rates in the general population.
- Breast cancer risk was highest among survivors of sarcoma (SIR, 5.3; 95% CI, 3.6–7.8) and leukemia (SIR, 4.1; 95% CI, 2.4–6.9), for whom the cumulative incidence of breast cancer was estimated to be 5.8% and 6.3%, respectively, by age 45 years.
- Treatment with alkylating agents and anthracyclines increased the risk of breast cancer in a dose-dependent manner.
- CCSS investigators also examined SN risk among 7,448 participants who were treated with chemotherapy only.[12]
- Breast cancer incidence was 4.6-fold greater than what would be expected in the general population (SIR, 4.6; 95% CI, 3.5–6.0).
- A linear dose response was demonstrated between anthracyclines and breast cancer rate (RR, 1.3/100 mg/m2; 95% CI, 1.2–1.6).
- DCOG-LATER investigators evaluated the contribution of chemotherapy to solid cancer risk in a large cohort of childhood cancer survivors diagnosed between 1963 and 2001.[13]
- Survivors treated with doxorubicin exhibited a dose-dependent increased risk of breast cancer (HR, 3.1; 95% CI, 1.4–6.5 among survivors treated with anthracycline doses of 250 mg/m2 or higher).
- The doxorubicin–breast cancer dose response was stronger for survivors of Li-Fraumeni–associated cancers (leukemia, CNS, and sarcomas other than Ewing) than for survivors of other cancers.
- A SEER Program–based study evaluated trends in surgical management and the 5- and 10-year cumulative incidence of contralateral breast cancer among women who were treated with radiation therapy for Hodgkin lymphoma before age 30 years and diagnosed with a subsequent breast cancer between 1990 and 2016.[45]
- Overall, 263 (89.2%) women presented with unilateral breast cancer, 50 of whom (19.0%) underwent breast-conserving surgery and 213 of whom (81.0%) underwent mastectomy (40.5% bilateral).
- The 5-year incidence of contralateral breast cancer in women who underwent unilateral surgery was 9.4% (95% CI, 5.6%–15.4%), increasing to 20.2% (95% CI, 13.7%–29.2%) at 10 years and 29.9% (95% CI, 20.8%–41.9%) at 15 years. This finding underscores the importance of ongoing breast cancer surveillance and consideration of prophylactic mastectomy.
Breast cancer risk varies among childhood cancer survivors who are treated with chest radiation therapy, and the risk is based on multiple clinical factors. The first personalized breast cancer risk prediction model was developed and validated using multinational cohorts of female 5-year cancer survivors who were diagnosed at younger than 21 years and treated with chest irradiation (n = 2,147). The model includes current age, chest radiation field, whether chest radiation was delivered within 1 year of menarche, anthracycline exposure, age of menopause, and history of a first-degree relative with breast cancer. The model is available as an online risk calculator.[46]
Subsequent versus de novo breast cancer
Several studies have investigated the clinical characteristics of subsequent breast cancers arising in women treated with radiation therapy for childhood cancer.[47,48,49,50,51]
- In one population-based study, radiation-induced breast cancer was noted to have more adverse clinicopathological features, as evidenced by a twofold increased risk of estrogen receptor–negative, progesterone receptor–negative breast cancer observed among 15-year Hodgkin lymphoma survivors, compared with women who had sporadic breast cancer.[47]
- Other studies have observed a higher proportion of more histologically aggressive subtypes (e.g., triple-negative breast cancer) than age-matched sporadic invasive cancers.[48,49]
- These findings are in contrast to other smaller, hospital-based, case-control studies of breast cancer among Hodgkin lymphoma survivors that have not identified a significant variation in hormone receptor status when compared with primary breast cancer controls. Previous studies have also not demonstrated a significant difference in overall risk of high-grade versus low-grade tumors.[50,51]
- A study using SEER Program data evaluated the clinicopathological features of 321 breast cancers diagnosed in 257 women (median age, 22 years; range, 18–26 years at Hodgkin lymphoma diagnosis) who were previously treated with radiation for Hodgkin lymphoma.[52]
- Women who developed breast cancer after radiation for Hodgkin lymphoma were younger than women diagnosed with breast cancer without a prior malignancy (43 vs. 60 years) and were less likely to present with ductal carcinoma in situ (0.4% vs. 14.9%).
- Radiation was not associated with poorer biological features, compared with breast cancers diagnosed in similarly aged women with no prior malignancy. However, higher proportions of upper outer quadrant and smaller tumors (≤2 cm) were found.
- Most tumors in survivors of Hodgkin lymphoma were hormone sensitive (nearly two-thirds were estrogen receptor–positive/HER2-negative). This finding suggests that endocrine prevention may be an option for this high-risk population.
Mortality after subsequent breast cancer
In a study of female participants in the CCSS who were subsequently diagnosed with breast cancer (n = 274) and matched to a control group of women (n = 1,095) with de novo breast cancer, the following was observed:[53]
- Survivors of childhood cancer were found to have elevated mortality rates (HR, 2.2; 95% CI, 1.7–3.0), even after adjusting for breast cancer treatment.
- Survivors were five times more likely to die from other health-related causes, including other SMNs and cardiovascular or pulmonary disease (HR, 5.5; 95% CI, 3.4–9.0).
- The cumulative incidence of a second asynchronous breast cancer was elevated significantly compared with controls (at 5 years, 8.0% among childhood cancer survivors vs. 2.7% among controls; P < .001).
Although current evidence does not show a survival benefit from the initiation of breast cancer surveillance in women treated with radiation therapy to the chest for childhood cancer, interventions to promote detection of small and early-stage tumors may improve prognosis. Those with more limited treatment options because of previous exposure to radiation or anthracyclines may especially benefit.
In support of surveillance, SJLIFE investigators observed that breast cancers detected by imaging and/or prophylactic mastectomy were more likely to be in situ carcinomas, be smaller masses (3.3 cm mean tumor size detected by physical examination vs. 1.1 cm detected by imaging), have no lymph node involvement, and be treated without chemotherapy, compared with breast cancers detected by physical findings.[24]
Investigators used data from the CCSS and two Cancer Intervention and Surveillance Modeling Network breast cancer simulation models to estimate the clinical benefits, harms, and cost-effectiveness of breast cancer screening among childhood cancer survivors who were previously treated with chest radiation.[54]
- Screening with mammography and MRI, as recommended in COG Long-Term Follow-Up guidelines, is projected to avert half of the expected breast cancer deaths among high-risk survivors.
- On an annual schedule, a survivor will have an average of 4 to 5 false-positive screening results and 1 to 2 benign biopsy results over the course of their lifetime.
- Because of the large survival benefits, the harm-benefit tradeoffs for survivors were found to be appropriate, resulting in more favorable harm-benefit ratios.
Another CCSS investigation quantified the association between temporal changes in cancer treatment over three decades and subsequent breast cancer risk.[35]
- The cumulative incidence of breast cancer was 8.1% (95% CI, 7.3%–9.0%) by age 45 years among 11,550 female survivors (median age, 34.2 years), representing a more than sixfold excess risk (SIR, 6.6; 95% CI, 6.1–7.2), compared with age-, sex-, and calendar year–matched controls.
- Concurrent with changes in therapy by decade, including reduced rates of chest and pelvic radiation therapy and increased rates of anthracycline chemotherapy exposure, and adjusting for age and age at diagnosis, the invasive breast cancer rate decreased 18% for every 5 years of primary cancer diagnosis era (rate ratio, 0.82; 95% CI, 0.74–0.90). This finding was largely associated with the reduced rate of chest radiation therapy.
Thyroid cancer
Thyroid cancer is observed after the following:[12,23,55,56]
- Neck radiation therapy for Hodgkin lymphoma, acute lymphoblastic leukemia (ALL), and brain tumors.
- Iodine I 131-metaiodobenzylguanidine (131I-MIBG) treatment for neuroblastoma.
- TBI for HSCT.
- Chemotherapy only, without therapeutic radiation.
The 25-year cumulative incidence of thyroid cancer among survivors of childhood cancer is 0.5%.[13] The risk of thyroid cancer among childhood cancer survivors is more than tenfold higher than that of the general population (SIR, 10.5; 95% CI, 9.1–12).[4] Significant modifiers of the radiation-related risk of thyroid cancer include the following:[27,57]
- Female sex.
- Younger age at exposure.
- Longer time since exposure.
- Radiation dose. A linear dose-response relationship between radiation exposure and thyroid cancer is observed up to 10 Gy, with a leveling off between 10 Gy and 30 Gy, and a decline in the OR at higher doses, especially in children younger than 10 years at treatment, suggesting a cell killing effect of the target cells at higher doses.[23,27,58]
In a Dutch case-control study, childhood cancer survivors with subsequent thyroid cancer were more likely to present with smaller tumors and bilateral tumors than the general population. Treatment outcomes were similar between subsequent and sporadic thyroid cancers.[59]
For information about detecting thyroid nodules and thyroid cancer, see the Thyroid nodules section.
CNS tumors
Subsequent CNS tumors represent a spectrum of histological subtypes, from high-grade gliomas to benign meningiomas. The CCSS has reported briefer latency for gliomas than for meningiomas.[60] Accurate assessment of the prevalence of low-grade and benign lesions is challenging because of the variable opinions and practices related to neuroimaging versus symptom surveillance in long-term survivors treated with cranial irradiation. Therefore, the prevalence of these tumors is likely higher than proven.
Brain tumors develop after cranial irradiation for histologically distinct brain tumors or for management of disease among ALL or non-Hodgkin lymphoma patients.[61] SIRs reported for subsequent CNS neoplasms after treatment for childhood cancer range from 8.1 to 52.3 across studies.[23]
The risk of subsequent brain tumors demonstrates a linear relationship with radiation dose.[25,60]
- The risk of meningioma after radiation increases with radiation dose,[9] and in some studies is further potentiated with increased exposure to intrathecal methotrexate.[25] However, this finding has not been consistently replicated.[10]
- Cavernomas have also been reported with considerable frequency after CNS irradiation but have been speculated to result from angiogenic processes as opposed to true tumorigenesis.[62,63,64]
The Dutch Long-Term Effects after Childhood Cancer (LATER) investigators have described the clinical characteristics of childhood cancer survivors who developed histologically confirmed meningiomas.[11]
- In 6,015 childhood cancer survivors from the LATER cohort, 1,551 of whom had prior cranial radiation therapy, 93 survivors developed meningiomas.
- Of these patients, 89 (95.7%) were treated with prior cranial radiation therapy. The median age at diagnosis was 31.8 years (range, 13.2–50.5 years).
- Thirty of the survivors presented with synchronous meningiomas, and 84 survivors presented with symptoms. Only 16% of the meningiomas were detected in late effects clinics.
- All survivors underwent surgery, and one-third (n = 31) of them also received radiation therapy. Twelve survivors had three or more surgeries for growth of residual tumor, recurrences, and new meningiomas. Although the extent of surgical resection was not described, the indications for radiation therapy during follow-up after surgical resection included residual tumor, recurrences, location, or new meningioma. During follow-up, 38 survivors (40.9%) developed new meningiomas, 22 (23.7%) had recurrences, and at least 4 died because of the meningioma.
Neurological sequelae associated with meningiomas can include seizures, auditory-vestibular-visual deficits, focal neurological dysfunction, and severe headaches.[9] Despite the well-established increased risk of subsequent CNS neoplasms among childhood cancer survivors treated with cranial irradiation and the growing recognition of associated morbidity, the current literature is insufficient to evaluate the potential harms and benefits of routine screening for these lesions.[65] The decision to initiate surveillance should be shared by the cancer survivor and health care provider after carefully considering the potential harms and benefits of surveillance for CNS neoplasms, such as meningioma.
Proton radiation therapy for pediatric medulloblastoma is associated with low rates of brain stem injury and secondary malignancies. The long-term effects were reported in 178 pediatric patients with medulloblastoma who were treated with surgery, proton radiation therapy, and chemotherapy between 2002 and 2016 (median follow-up, 9.3 years).[66]
- At 10 years, eight patients (4.5%) developed a secondary tumor (benign or malignant), with median follow up of 9.1 years. Three patients (1.7%) developed a secondary malignancy.
- In-field second malignancies were glioblastoma (n = 2), meningioma (n = 2), and high-grade glioma (n = 1). The other malignancies were one each of ovarian fibroma, plexiform fibromyxoma of the esophagus, and papillary thyroid carcinoma.
- Four patients developed brain stem injury at a median of 4.2 years. The 5-year cumulative incidence of brain stem injury was 1.1%, and the 10-year cumulative incidence was 1.9%.
Bone and soft tissue tumors
Survivors of hereditary retinoblastoma, Ewing sarcoma, and other malignant bone tumors are at a particularly increased risk of developing subsequent bone and soft tissue tumors.[67,68,69,70,71]
- Radiation therapy is associated with a linear dose-response relationship.[72,73]
- After adjustment for radiation therapy, treatment with alkylating agents [13] and anthracyclines [74] have both been linked to sarcoma, with the risk increasing with cumulative drug exposure.[74]
- Soft tissue sarcomas can be of various histological subtypes, including nonrhabdomyosarcoma soft tissue sarcomas, rhabdomyosarcoma, malignant peripheral nerve sheath tumors, Ewing/primitive neuroectodermal tumors, and other rare tumor types.
Evidence (excess risk of bone and soft tissue tumors):
- A nested case-control study included 228 cases and 228 matched controls within a cohort of 69,460 5-year survivors of childhood cancer. The study investigated the risks of subsequent primary bone cancer by different levels of cumulative radiation exposure and dose-response relationships according to different specific types of chemotherapy.[75]
- Compared with unexposed bone tissue, the OR associated with bone tissue exposed to 1 to 4 Gy was 4.8-fold (95% CI, 1.2–19.6). The OR associated with bone tissue exposed to 5 to 9 Gy was 9.6-fold (95% CI, 2.4–37.4). The OR increased linearly with increasing doses of radiation (Ptrend < .001), up to 78-fold (95% CI, 9.2–669.9) for doses of 40 Gy or higher.
- For cumulative alkylating agent doses of 10,000 to 19,999 mg/m2 and 20,000 mg/m2 or higher, the radiation-adjusted ORs were 7.1 (95% CI, 2.2–22.8) and 8.3 (95% CI, 2.8–24.4), respectively. There were independent contributions from exposure to procarbazine, ifosfamide, or cyclophosphamide.
- A population-based study of 69,460 5-year survivors of cancer diagnosed before age 20 years observed the following:[68,69]
- The risk of subsequent primary bone cancer was 22-fold greater than that of the general population, with an estimated 45-year cumulative incidence of 0.6%, compared with an expected rate of 0.03% in the general population.[68]
- The observed excess numbers of subsequent primary bone cancer declined with both age and years from diagnosis.[68]
- The risk of subsequent soft tissue sarcoma was almost 16-fold higher than the general population, with an estimated 45-year cumulative incidence of 1.4%, compared with an expected rate of 0.1%.[69]
- The median time from diagnosis to occurrence of a soft tissue sarcoma was 19 years.[69]
- The most commonly observed soft tissue sarcomas were leiomyosarcoma, fibromatous neoplasms, and malignant peripheral nerve sheath tumors.[69]
- The SIR for subsequent fibromatous primary sarcomas decreased with increasing years from diagnosis and attained age, whereas the SIR for leiomyosarcoma and malignant peripheral nerve sheath tumors remained consistently high across all years from diagnosis and at all attained ages.[69]
- The absolute excess risks of all sarcoma subtypes were generally low, except for leiomyosarcoma that followed a retinoblastoma diagnosis (absolute excess risks, 52.7 per 10,000 person-years among survivors 45 years or more from diagnosis).[69]
- The risk of developing a leiomyosarcoma was 30-fold higher among survivors of childhood cancer, compared with an excess risk of 0.7 for the general population.[69]
- Retinoblastoma survivors were at the highest risk (SIR, 342.9), followed by Wilms tumor survivors (SIR, 74.2).
- 90% of leiomyosarcomas observed after a Wilms tumor diagnosis developed within the irradiated tissue.
- In a CCSS cohort, an increased risk of subsequent bone or soft tissue sarcoma was associated with radiation therapy, a primary diagnosis of sarcoma, a history of other SNs, and treatment with higher doses of anthracyclines or alkylating agents.[26]
- The 30-year cumulative incidence of subsequent sarcoma in CCSS participants was 1.08% for survivors who received radiation therapy and 0.5% for survivors who did not receive radiation therapy.
- Dose-risk modeling was used to study the risk of bone sarcoma in a retrospective cohort of 4,171 survivors of a childhood solid cancer treated between 1942 and 1986 (median follow-up, 26 years).[72]
- Results demonstrated that the risk of bone sarcoma increased slightly up to a cumulative organ-absorbed radiation dose of 15 Gy (HR, 8.2; 95% CI, 1.6–42.9) and then rapidly increased for higher radiation doses (HR for 30 Gy or more, 117.9; 95% CI, 36.5–380.6), compared with patients not treated with radiation therapy.
- The excess RR per Gy in this model was 1.77 (95% CI, 0.62–5.94).
- In survivors of bilateral retinoblastoma, the most commonly observed malignant SN is sarcoma, specifically osteosarcoma.[76,77,78] The contribution of chemotherapy to solid malignancy carcinogenesis was highlighted in a long-term follow-up study of 906 5-year hereditary retinoblastoma survivors who were diagnosed between 1914 and 1996 and observed through 2009.[67]
- Treatment with alkylating agents significantly increased risk of subsequent bone tumors (HR, 1.60; 95% CI, 1.03–2.49) and leiomyosarcoma (HR, 2.67; 95% CI, 1.22–5.85) among members of the cohort.
- Leiomyosarcoma occurrence was more common after treatment with alkylating agent chemotherapy and radiation therapy compared with radiation therapy alone (5.8% vs. 1.6% at age 40 years; P = .01).
- The CCSS reported the following on 105 cases and 422 matched controls in a nested case-control study of 14,372 childhood cancer survivors:[74]
- Soft tissue sarcomas occurred at a median of 11.8 years (range, 5.3–31.3 years) from original diagnoses.
- Any exposure to radiation was associated with increased risk of soft tissue sarcoma (OR, 4.1; 95% CI, 1.8–9.5), which demonstrated a linear dose-response relationship.
- Anthracycline exposure was associated with soft tissue sarcoma risk (OR, 3.5; 95% CI, 1.6–7.7), independent of radiation dose.
- In a cohort of 952 irradiated survivors of hereditary retinoblastoma diagnosed between 1914 and 2006, CCSS investigators observed that elevated bone and soft tissue sarcoma risks differed by age, location, and sex.[78]
- Head and neck bone and soft tissue sarcomas were diagnosed beginning in early childhood and continued well into adulthood (60-year cumulative incidence of 6.8% and 9.3%, respectively).
- Body and extremity bone sarcoma incidence flattened after adolescence (60-year cumulative incidence, 3.5%).
- Body and extremity soft tissue sarcoma incidence was rare until age 30 years, when incidence rose steeply (60-year cumulative incidence, 6.6%) particularly for females (60-year cumulative incidence, 9.4%).
- In a retrospective study of 160 patients with hereditary retinoblastoma who received radiation therapy, no correlation was identified between age (before or after 12 months) at which external-beam radiation therapy was given and development of subsequent malignancy.[70]
- Patients with and without subsequent malignancies did not differ by RB1 variant type. Also, there was no association with variant type and location of SMN, or SMN type and age at diagnosis.
- The study showed that patients who have a low penetrance variant and receive external-beam radiation therapy remain at risk of SMNs and should be cautiously monitored.
Skin cancer
Nonmelanoma skin cancers (NMSCs) are one of the most common SNs among childhood cancer survivors and show a strong association with radiation therapy.[4,79] Adherence to sun protection behaviors can reduce exposure to UV radiation that may exacerbate risk.
The CCSS performed a randomized, controlled, comparative effectiveness trial to test methods to improve early detection of skin cancer among survivors of childhood cancer at high risk after radiation therapy exposure. Participants were randomly assigned to the experimental arm, which included print materials in combination with mHealth strategies (text messages and use of the Advancing Survivors' Knowledge website), or the control arm. Screening rates improved by 1.5-fold in the experimental arm. Rates of physician skin examination increased from baseline to 12 months, and rates of self-examination increased from baseline to 18 months in all three intervention groups. However, the increase in rates did not differ between the intervention groups.[80]
Evidence (excess risk of NMSCs):
- Compared with participants who did not receive radiation therapy, CCSS participants treated with radiation therapy had a 6.3-fold increased risk of NMSCs (95% CI, 3.5–11.3).[81]
- 90% of tumors occurred within the radiation field.
- A CCSS case-control study of the same cohort reported on subsequent basal cell carcinomas (BCCs). Children who received 35 Gy or more to the skin site had an almost 40-fold excess risk of developing BCCs (OR, 39.8; 95% CI, 8.6–185), compared with those who did not receive radiation therapy. Results were consistent with a linear dose-response relationship, with an excess OR per Gy of 1.09 (95% CI, 0.49–2.64).
- In 5,843 childhood cancer survivors in the DCOG-LATER cohort, investigators found that childhood cancer survivors had a 30-fold increased risk of developing BCCs.[29]
- After a first BCC diagnosis, 46.7% of patients developed additional BCCs.
- BCC risk was associated with any radiation therapy to the relevant radiation field (site of BCC) (HR, 14.32) and with estimated percentage of exposed skin surface area (26%–75%: HR, 1.99; 76%–100%: HR, 2.16 vs. 1%–25% exposed; Ptrend among exposed = .002).
- BCC risk was not associated with prescribed radiation dose and likelihood of sun-exposed skin area.
- Of all chemotherapy groups examined, only vinca alkaloids increased the BCC risk (HR, 1.54).
- The occurrence of an NMSC as the first SN has been reported to identify a population at high risk of a future invasive malignant SN.[7]
- CCSS investigators observed a cumulative incidence of a malignant neoplasm of 20.3% (95% CI, 13.0%–27.6%) at 15 years among radiation-exposed survivors who developed an NMSC as a first SN, compared with 10.7% (95% CI, 7.2%–14.2%) among survivors whose first SN was an invasive malignancy.
Malignant melanoma has also been reported as an SN in childhood cancer survivor cohorts, although at a much lower incidence than NMSCs.
Risk factors for malignant melanoma identified among these studies include the following:[82]
- Radiation therapy.
- Combination of alkylating agents and antimitotic drugs.
Evidence (excess risk of melanoma):
- A systematic review that included data from 19 original studies (total N = 151,575 survivors; median follow-up of 13 years) observed an incidence of 10.8 cases of malignant melanoma per 100,000 childhood cancer survivors per year.[82]
- Melanomas most frequently developed in survivors of Hodgkin lymphoma, hereditary retinoblastoma, soft tissue sarcoma, and gonadal tumors. However, the relatively small number of survivors represented in the relevant studies preclude assessment of melanoma risk among other types of childhood cancer.
- CCSS investigators observed an approximate 2.5-fold increased risk (SIR, 2.42; 95% CI, 1.77–3.23) of melanoma among members of their cohort (median time to development, 21 years).[83]
- The cumulative incidence of first subsequent melanoma at 35 years from initial cancer diagnosis was 0.55% (95% CI, 0.37%–0.73%), and the absolute excess risk was 0.10 per 1,000 person-years (95% CI, 0.05–0.15).
- Family history of cancer, demographic characteristics, or treatment-related factors did not predict risk of melanoma.
Skin cancer risk after retinoblastoma
The incidence of melanoma and NMSC was evaluated in a cohort of 1,851 long-term, White survivors of retinoblastoma (1,020 hereditary and 831 nonhereditary) who were diagnosed from 1914 to 2006 and monitored through 2016.[84]
- Of all patients, 33 hereditary and 7 nonhereditary survivors developed melanoma, and 26 hereditary and 9 nonhereditary survivors developed NMSC. The median age of skin cancer development was about 20 years younger for hereditary survivors than nonhereditary survivors.
- Most NMSCs were on the head/neck, whereas melanomas were more broadly distributed, with patterns similar to melanoma-prone families.
- At 50 years after retinoblastoma diagnosis, in hereditary survivors, the cumulative incidence of melanoma was 4.5% and the cumulative incidence of NMSC was 3.7%. In nonhereditary survivors, the cumulative incidence of melanoma was 0.7% and the cumulative incidence of NMSC was 1.5%.
Lung cancer
Among childhood cancer survivor cohorts, lung cancer represents a relatively uncommon SN. There is a long latent period between the childhood cancer diagnosis and the development of a lung SN.[13,85]
Evidence (excess risk of lung cancer):
- The 30-year cumulative incidence of lung cancer among CCSS participants was 0.16% (95% CI, 0.09%–0.23%), representing a SIR of 4.0 (95% CI, 2.9–5.4).[85]
- Lung cancer risk demonstrated a dose-related association with chest radiation dose: 10 to 30 Gy (HR, 3.4; 95% CI, 1.05–11.0), more than 30 to 40 Gy (HR, 4.6; 95% CI, 1.5–14.3), and more than 40 Gy (HR, 9.1; 95% CI, 3.1–27.0).
- Cumulative incidence of lung SMNs was higher among current or past smokers, compared with those who had never smoked.
- The SIR for lung cancer was highest among survivors of Hodgkin lymphoma (SIR, 9.3; 95% CI, 6.2–13.4) and bone cancer (SIR, 4.4; 95% CI, 1.8–9.1).
- The 25-year cumulative incidence of lung cancer among the DCOG-LATER cohort was 0.1% (95% CI, 0.0%–0.3%).[13]
- Incidence was approximately fourfold higher than what would be expected in the general population (SIR, 4.3; 95% CI, 1.9–8.5).
- Lung cancer has been reported after chest irradiation for Hodgkin lymphoma.[86]
- The risk increases in association with longer elapsed time from diagnosis.
- Smoking has been linked with the occurrence of lung cancer that develops after radiation therapy for Hodgkin lymphoma.[86]
- The increase in risk of lung cancer with increasing radiation dose is greater among patients who smoke after exposure to radiation than among those who refrain from smoking (P = .04).
Gastrointestinal (GI) cancer
There is substantial evidence that childhood cancer survivors develop GI malignancies more frequently and at a younger age than the general population. This evidence supports the need for early initiation of colorectal carcinoma surveillance.[87,88,89]
Evidence (excess risk of GI cancer):
- In a French and British cohort-nested, case-control study of childhood solid tumor survivors diagnosed before age 17 years, the risk of developing a digestive organ SN varied with therapy.[90]
- The risk of GI cancer was 9.7-fold higher than in population controls.
- The SNs most often involved the colon/rectum (42%), liver (24%), and stomach (19%).
- A strong radiation dose-response relationship was observed, with an OR of 5.2 (95% CI, 1.7–16.0) for local radiation doses between 10 Gy to 29 Gy and 9.6 (95% CI, 2.6–35.2) for doses of 30 Gy and above, compared with survivors who had not received radiation therapy.
- Chemotherapy alone and combined-modality therapy were associated with a significantly increased risk of developing a GI SN (SIR, 9.1; 95% CI, 2.3–23.6; SIR 29.0; 95% CI, 20.5–39.8, respectively).
- The PanCare Childhood and Adolescent Cancer Survivor Care and Follow-Up Studies (PanCareSurFup) consortium quantified the absolute risks by radiation therapy treatment characteristics in a cohort of 69,450 5-year childhood cancer survivors in Europe. ORs were calculated from a case-control study comprising 143 subsequent colorectal cancers within the cohort of childhood cancer survivors.[89]
- Survivors treated with any abdominopelvic radiation therapy were three times more likely to develop a subsequent colorectal cancer than those who did not receive radiation therapy (OR, 3.1; 95% CI, 1.4–6.6).
- By age 40 years, survivors who were treated with abdominopelvic radiation therapy already have a similar risk of colorectal cancer as individuals aged 50 years in the general population when population-based colorectal cancer screening begins.
- The risk of colorectal cancer increases with abdominopelvic radiation therapy dose. Survivors treated with spinal or whole-abdomen radiation therapy are at higher risk than those treated with radiation to other abdominopelvic fields.
- CCSS investigators reported a 4.6-fold higher risk of GI SNs among their study participants than in the general population (95% CI, 3.4–6.1).[87]
- The SNs most often involved the colon (39%), rectum/anus (16%), liver (18%), and stomach (13%).
- The SIR for colorectal cancer was 4.2 (CI, 2.8–6.3).
- The most prevalent GI SN histology was adenocarcinoma (56%).
- The highest risk of GI SNs was associated with abdominal irradiation (SIR, 11.2; CI, 7.6–16.4), but survivors not exposed to radiation also had a significantly increased risk (SIR, 2.4; CI, 1.4–3.9).
- High-dose procarbazine (RR, 3.2; CI, 1.1–9.4) and platinum drugs (RR, 7.6; CI, 2.3–25.5) independently increased the risk of GI SNs.
- St. Jude Children's Research Hospital investigators observed that the SIR for subsequent colorectal carcinoma was 10.9 (95% CI, 6.6–17.0) compared with U.S. population controls. Investigators also observed the following:[88]
- Incidence of a subsequent colorectal carcinoma increased steeply with advancing attained age, with a 40-year cumulative incidence of 1.4% ± 0.53% among the entire cohort (N = 13,048) and 2.3% ± 0.83% for 5-year survivors.
- Colorectal carcinoma risk increased by 70% with each 10 Gy increase in radiation dose. Increasing radiation volume also increased the risk.
- Treatment with alkylating agent chemotherapy was also associated with an 8.8-fold excess risk of subsequent colorectal carcinoma.
- A multi-institutional prospective study observed that potentially precancerous neoplastic polyps were found in 27.8% of childhood cancer survivors who received radiation to the abdomen/pelvis at least 10 years earlier and who had colonoscopic screening between age 35 and 49 years.[91]
- This polyp prevalence is at least as high as that previously reported for the average-risk population older than 50 years and is similar to the 24% incidence rate for patients with hereditary nonpolyposis colon cancer. Polyp prevalence rates in the general population for people aged 35 to 49 years are unclear.
- A DCOG-LATER record linkage study evaluated the risk of histologically confirmed colorectal adenomas among 5,843 5-year childhood cancer survivors followed for a median of 24.9 years.[92]
- The cumulative incidence of colorectal adenoma by age 45 years was 3.6% among survivors who received abdominal pelvic radiation versus 2.0% for survivors who did not receive abdominal pelvic radiation, versus 1.0% among siblings.
- Factors associated with adenoma risk were abdominal pelvic radiation (HR, 2.1), TBI (HR, 10.6), cisplatin (HR, 2.1 for <480 mg/m2; HR, 3.8 for ≥480 mg/m2), diagnosis of hepatoblastoma (HR, 27.1), and family history of early-onset colorectal cancer (HR, 20.5).
- Procarbazine exposure was also associated with an increased risk among survivors not exposed to abdominal pelvic radiation or TBI (HR, 2.7).
- The PanCareSurFup consortium reported on digestive cancers in a cohort of 69,460 5-year childhood cancer survivors in Europe.[93]
- Survivors of Wilms tumor (SIR, 12.1; 95% CI, 9.6–15.1) and Hodgkin lymphoma (SIR, 7.3; 95% CI, 5.9–9.0) were at the highest risk for GI SMNs.
- By age 55 years, 2.3% of survivors of Wilms tumor and Hodgkin lymphoma developed a secondary colorectal cancer, a rate that is comparable to that of the general population with two or more first-degree relatives affected by GI cancer.
- A nested case-control study examined the rate of colorectal cancer according to large bowel radiation therapy dose and procarbazine dose among 5-year Hodgkin lymphoma survivors who were diagnosed between age 15 and 50 years (only 27% of cases were 15 to 24 years old) at five hospital centers in the Netherlands (diagnosed between 1964 to 2000; median follow-up, 26 years).[94]
- The median interval between Hodgkin lymphoma diagnosis and subsequent colorectal cancer was 25.7 years (range, 18.2–31.6 years).
- Treatment with subdiaphragmatic radiation therapy (RR, 2.4; 95% CI, 1.4–4.1) and more than 8.4 g/m2 of procarbazine (RR, 2.5; 95% CI, 1.3–5.0) were associated with increased rates of colorectal cancer on univariable analysis.
- Colorectal cancer rate increased linearly with mean radiation therapy dose to the whole large bowel and dose to the affected bowel segment. The dose response became steeper with higher doses of procarbazine and increased 1.2-fold (95% CI, 1.1–1.3) for each 1 g/m2 increase in procarbazine.
Oral cancers
The PanCareSurFup consortium reported on risks of oral second primary neoplasms (validated through pathology reports) in a cohort of 69,460 5-year childhood cancer survivors in Europe.[95]
- Oral second primary neoplasms (n = 145; 64 salivary gland, 38 tongue, 20 pharynx, 2 lip, and 21 other) developed in 143 survivors at a median age of 32 years. This represents a fivefold excess risk compared with that expected in the general population.
- Childhood cancer diagnostic groups at greatest risk for an oral second primary neoplasm included leukemia (SIR, 19.2; 95% CI, 14.6–25.2), bone sarcoma (SIR, 6.4; 95% CI, 3.7–11.0), Hodgkin lymphoma (SIR, 6.2; 95% CI, 3.9–9.9), and soft tissue sarcoma (SIR, 5.0; 95% CI, 3.0–8.5).
- Observed specific treatment associations with oral second primary neoplasms included radiation therapy and salivary gland neoplasms (SIR, 33; 95% CI, 25.3–44.5) and chemotherapy (any exposure) and tongue neoplasms (SIR, 15.9; 95% CI, 10.6–23.7).
- Data were not available to assess the contributions of health behaviors (smoking, alcohol intake) and human papillomavirus (HPV) infection status to the development of oral second primary neoplasms.
Urogenital cancers
Development of subsequent primary urogenital cancers in childhood and adolescent cancer survivors is rare.
Using SEER data of 43,991 patients (aged <20 years) diagnosed with a first primary cancer from 1975 to 2016, the risk of urinary system cancer was higher for both females (SIR, 5.18; 95% CI, 3.65–7.14) and males (SIR, 2.80; 95% CI, 1.94–3.92), compared with the general population.[96]
- Females were more likely than males to develop a subsequent urinary system cancer (SIR, 1.86; 95% CI, 1.13–3.03) and kidney cancer (SIR, 1.97; 95% CI, 1.11–3.53).
- Females with any first cancer had higher risks than the general population for developing cancers of the corpus uteri (SIR, 2.32; 95% CI, 1.49–3.45) and vulva (SIR, 4.27; 95% CI, 1.38–9.95).
Renal carcinoma
Consistent with reports among survivors of adult-onset cancer, an increased risk of renal carcinoma has been observed in survivors of childhood cancer.[28,97,98] Underlying genetic predisposition may also play a role in the risk of developing renal carcinomas because rare cases of renal carcinoma have been observed in children with tuberous sclerosis.[97] Cases of secondary renal carcinoma associated with Xp11.2 translocations and TFE3 gene fusions have also been reported and suggest that cytotoxic chemotherapy may contribute to renal carcinogenesis.[99,100,101]
Evidence (excess risk of renal carcinoma):
- CCSS investigators reported a significant excess of subsequent renal carcinoma among 14,358 5-year survivors in the cohort (SIR, 8.0; 95% CI, 5.2–11.7) compared with the general population.[97]
- The reported overall absolute excess risk of 8.4 per 105 person-years indicates that these cases are relatively rare. Highest risk was observed among the following:
- Neuroblastoma survivors (SIR, 85.8; 95% CI, 38.4–175.2).[97] Radiation has been hypothesized to predispose children with high-risk neuroblastoma to renal carcinoma.[102]
- Those treated with renal-directed radiation therapy of 5 Gy or higher (RR, 3.8; 95% CI, 1.6–9.3).[97]
- Those treated with platinum-based chemotherapy (RR, 3.5; 95% CI, 1.0–11.2).[97]
Human Papillomavirus (HPV)–Associated Malignancies
Evidence (HPV-associated SMNs):
- A CCSS study evaluated the occurrence of cancer types in which HPV is an established etiologic risk factor. The study included 24,363 childhood cancer survivors who were a median of 21 years from diagnosis.[103]
- The 30-year cumulative incidence of an HPV-associated cancer was 0.3% (95% CI, 0.2%–0.4%), reflecting an almost threefold excess risk (SIR, 2.86; 95% CI, 2.05–4.00) to that of the general population.
- Male and female cancer survivors had an elevated risk of HPV-associated oropharyngeal (SIR in males, 4.06; SIR in females, 8.44) and anorectal (SIR in males, 13.56; SIR in females, 9.16) SMNs. However, females were not at increased risk of cervical or vulvar cancers, compared with the general population.
- Independent risk factors for HPV-associated cancers identified by multivariable modeling included male sex (vs. females: relative SIR, 1.99; 95% CI, 1.00–3.94); head, neck, and pelvic radiation therapy doses higher than 30 Gy (vs. none: relative SIR, 2.35; 95% CI, 1.11–4.97); and cisplatin-equivalent doses higher than 400 mg/m2 (vs. none: relative SIR, 4.51; 95% CI, 1.78–11.43).
- A population-based study used Surveillance, Epidemiology, and End Results (SEER) registry data to evaluate risk factors and trends for HPV-associated SMNs among 374,408 adolescent and young adult (AYA) patients (11% were aged 15–24 years, 28% were aged 25–34 years, and 61% were aged 35–44 years at diagnosis). The patients were diagnosed between 1976 and 2015.[104]
- The incidence of HPV-associated SMNs declined over the study period, and the overall incidence of HPV-associated SMNs among AYA cancer survivors was low, affecting only 0.4% of survivors.
- Risk of any HPV-associated SMN was increased by 70% for AYA survivors (SIR, 1.70; 95% CI, 1.61–1.79) and 117% for oropharyngeal cancer (SIR, 2.17; 95% CI, 2.00–2.35), compared with the general population.
- Cervical cancer risk was overall lower in survivors (SIR, 0.85; 95% CI, 0.76–0.95), but Hispanic AYA survivors had a significant increase in cervical cancer (SIR, 1.46; 95% CI, 1.01–2.06).
- Among survivors with HPV-related first cancers, prior chemotherapy and radiation therapy were associated with any HPV-related SMN. However, those therapies were not associated with HPV-related SMNs among survivors whose first cancers were not HPV related.
Survival Outcomes After SNs
Outcome after the diagnosis of an SN is variable, as treatment for some histological subtypes may be compromised if childhood cancer therapy included cumulative doses of agents and modalities at the threshold of tissue tolerance.
Using data from the SEER Program, individuals younger than 60 years with first primary malignancies (n = 1,332,203) were compared with childhood cancer survivors (n = 1,409) who had a second primary malignancy.[105]
- Survivors of childhood cancer diagnosed with a second primary malignancy experienced poorer overall survival than did their peers without a history of cancer (HR, 1.86; 95% Cl, 1.72–2.02) after the study had accounted for cancer type, age, sex, race, and decade of diagnosis.
- A history of childhood cancer was consistently associated with a twofold to threefold increased risk of death for the most diagnosed second primary malignancies, including breast cancer, thyroid cancer, AML, brain cancer, melanoma, bone cancer, and soft tissue sarcoma.
In a study of female participants in the CCSS who were subsequently diagnosed with breast cancer (n = 274) and matched to a control group of women (n = 1,095) with de novo breast cancer, survivors of childhood cancer were found to have elevated mortality rates (HR, 2.2; 95% CI, 1.7–3.0) even after adjusting for breast cancer treatment.[53]
- Survivors were five times more likely to die as a result of other health-related causes, including other SMNs and cardiovascular or pulmonary disease (HR, 5.5; 95% CI, 3.4–9.0).
- The cumulative incidence of a second asynchronous breast cancer was elevated significantly compared with controls (at 5 years, 8.0% among childhood cancer survivors vs. 2.7% among controls; P < .001).
Subsequent Neoplasms and Genetic Susceptibility
Literature clearly supports the role of chemotherapy and radiation therapy in the development of SNs. However, interindividual variability exists, suggesting that genetic variation has a role in susceptibility to genotoxic exposures, or that genetic susceptibility syndromes confer an increased risk of cancer, such as Li-Fraumeni syndrome.[106,107] In a population-based Swiss Childhood Cancer Survivor Study, cancer predisposition syndromes were associated with a high risk of second primary neoplasms before the age of 21 years and represented the most important risk factor (HR, 7.8; 95% CI, 4.8–12.7) for developing a second primary cancer.[108]
Previous studies have demonstrated that childhood cancer survivors with a family history of Li-Fraumeni syndrome in particular, or a family history of cancer, carry an increased risk of developing an SN.[109,110] A prospective registry followed 480 individuals with pathogenic or likely pathogenic germline TP53 variants.[111] Individuals who developed a first cancer were monitored for the development of a second malignant neoplasm. Among individuals who were younger than 17 years at the time of diagnosis of their first cancer, 50% developed a second cancer within 20 years.
The risk of SNs could potentially be modified by variants in high-penetrance genes that lead to these serious genetic diseases (e.g., Li-Fraumeni syndrome).[110] However, the attributable risk is expected to be very small because of the extremely low prevalence of variants in high-penetrance genes.
Likewise, children with neurofibromatosis type 1 (NF1) who develop a primary tumor are at an increased risk of SNs compared with childhood cancer survivors without NF1. Treatment with radiation, but not alkylating agents, increases the risk of SNs in survivors with NF1.[112] SNs represent a major contributor to excess mortality in adult survivors of childhood glioma with NF1.[113] These survivors developed late-onset (>5 years from diagnosis) SMNs at four times the rate of glioma survivors without NF1 (4.02; range, 2.12–7.62). The 30-year, all-cause late mortality rate was 46.3% (95% CI, 23.9%–62.2%) in glioma survivors with NF1, compared with 18% (95% CI, 16.1%–20.0%) in glioma survivors without NF1. The most common causes of death among survivors with NF1 and glioma were SNs.
Table 1 summarizes the spectrum of neoplasms, affected genes, and Mendelian mode of inheritance of selected syndromes of inherited cancer predisposition.
Table 1. Selected Syndromes of Inherited Cancer Predispositiona
Syndrome |
Major Tumor Types |
Affected Gene |
Mode of Inheritance |
AML = acute myeloid leukemia; MDS = myelodysplastic syndromes; WAGR = Wilms tumor, aniridia, genitourinary abnormalities, and range of developmental delays. |
a Adapted from Strahm et al.[114] |
b Dominant in a fraction of patients, spontaneous variants can occur. |
Adenomatous polyposis of the colon |
Colon, hepatoblastoma, intestinal cancers, stomach, thyroid cancer |
APC |
Dominant |
Ataxia-telangiectasia |
Leukemia, lymphoma |
ATM |
Recessive |
Beckwith-Wiedemann syndrome |
Adrenal carcinoma, hepatoblastoma, rhabdomyosarcoma, Wilms tumor |
CDKN1C,NSD1 |
Dominant |
Bloom syndrome |
Leukemia, lymphoma, skin cancer |
BLM |
Recessive |
Diamond-Blackfan anemia |
Colon cancer, osteogenic sarcoma, AML/MDS |
RPS19and otherRPgenes |
Dominant, spontaneousb |
Fanconi anemia |
Gynecological tumors, leukemia, squamous cell carcinoma |
FANCA, FANCB, FANCC, FANCD2, FANCE, FANCF, FANCG |
Recessive |
Juvenile polyposis syndrome |
Gastrointestinal tumors |
SMAD4 |
Dominant |
Li-Fraumeni syndrome |
Adrenocortical carcinoma, brain tumor, breast carcinoma, leukemia, osteosarcoma, soft tissue sarcoma |
TP53 |
Dominant |
Multiple endocrine neoplasia 1 |
Pancreatic islet cell tumor, parathyroid adenoma, pituitary adenoma |
MEN1 |
Dominant |
Multiple endocrine neoplasia 2 |
Medullary thyroid carcinoma, pheochromocytoma |
RET |
Dominant |
Neurofibromatosis type 1 |
Neurofibroma, optic pathway glioma, peripheral nerve sheath tumor |
NF1 |
Dominant |
Neurofibromatosis type 2 |
Vestibular schwannoma |
NF2 |
Dominant |
Nevoid basal cell carcinoma syndrome |
Basal cell carcinoma, medulloblastoma |
PTCH |
Dominant |
Peutz-Jeghers syndrome |
Intestinal cancers, ovarian carcinoma, pancreatic carcinoma |
STK11 |
Dominant |
Retinoblastoma |
Osteosarcoma, retinoblastoma |
RB1 |
Dominant |
Tuberous sclerosis |
Hamartoma, renal angiomyolipoma, renal cell carcinoma |
TSC1,TSC2 |
Dominant |
von Hippel-Lindau syndrome |
Hemangioblastoma, pheochromocytoma, renal cell carcinoma, retinal and central nervous system tumors |
VHL |
Dominant |
WAGR syndrome |
Gonadoblastoma, Wilms tumor |
WT1 |
Dominant |
Wilms tumor syndrome |
Wilms tumor |
WT1 |
Dominant |
Xeroderma pigmentosum |
Leukemia, melanoma |
XPA, XPB, XPC, XPD, XPE, XPF, XPG, POLH |
Recessive |
The McGill Interactive Pediatric OncoGenetic Guidelines (MIPOGG) tool identifies children with cancer who have an increased likelihood of having a cancer predisposition syndrome. This tool guides clinicians through a series of yes or no questions, and it generates a recommendation for or against genetic evaluation.[115,116]
- In a population-based, nested, case-control study, the MIPOGG tool identified survivors who were at increased risk of developing SMNs, after controlling for radiation and chemotherapy exposures (HR, 1.53; 95% CI, 1.06–2.19).
- SMN prediction was superior in survivors of CNS and solid tumor neoplasms and in survivors who were not irradiated during treatment of their primary malignancy.
- Use of the MIPOGG tool in pediatric oncology patients, at diagnosis or in survivorship follow-up, may help prioritize those who should undergo genetic evaluation.
Drug-metabolizing enzymes and DNA repair polymorphisms
The interindividual variability in risk of SNs is more likely related to common polymorphisms in low-penetrance genes that regulate the availability of active drug metabolites or are responsible for DNA repair. Gene-environment interactions may magnify subtle functional differences resulting from genetic variations.
In related research, SJLIFE investigators evaluated cancer treatments and pathogenic germline variants in 127 genes from six major DNA repair pathways to identify childhood cancer survivors at an increased risk of SNs.[117]
Drug-metabolizing enzymes
Metabolism of genotoxic agents occurs in two phases.
- Phase I involves activation of substrates into highly reactive electrophilic intermediates that can damage DNA, a reaction principally performed by the cytochrome p450 (CYP) family of enzymes.
- Phase II enzymes (conjugation) function to inactivate genotoxic substrates. The phase II proteins comprise the glutathione S-transferase (GST) enzymes, NAD(P)H:quinone oxidoreductase-1 (NQO1) enzyme, and others.
The balance between the two sets of enzymes is critical to the cellular response to xenobiotics; for example, high activity of a phase I enzyme and low activity of a phase II enzyme can result in DNA damage.
DNA repair polymorphisms
DNA repair mechanisms protect somatic cells from variants in tumor suppressor genes and oncogenes that can lead to cancer initiation and progression. An individual's DNA repair capacity appears to be genetically determined.[118] A number of DNA repair genes contain polymorphic variants, resulting in large interindividual variations in DNA repair capacity.[118] Evaluation of the contribution of polymorphisms influencing DNA repair to the risk of SN represents an active area of research.
Polygenic risk
With the decreased use of radiation therapy, it has become important to define the role of genetic susceptibility in chemotherapy-related SMNs. SJLIFE cohort study investigators evaluated treatment-related SMNs among long-term survivors of childhood cancer. An externally validated 179-variant polygenic risk score (PRS) associated with risks of common adult-onset cancers in the general population was calculated for each survivor.[119]
- The most frequent SMNs developing in 1,594 survivors included basal cell carcinoma (n = 822), breast cancer (n = 235), and thyroid cancer (n = 221).
- SMN risk associations with the PRS were extremely modest in survivors with European ancestry who were exposed to radiation therapy (HR, 1.22; n = 4,630).
- Among survivors with European ancestry who did not receive radiation therapy (n = 4,322), the increase in 30-year SMN cumulative incidence and HRs comparing top and bottom PRS quintiles was statistically significant for those treated with alkylating agents (17% vs. 6%; HR, 2.46; P < .01), anthracyclines (20% vs. 8%; HR, 2.86; P < .001), epipodophyllotoxins (23% vs. 1%; HR, 12.20; P < .001), or platinums (46% vs. 7%; HR, 8.58; P < .01).
- Among survivors with African ancestry who did not receive radiation therapy (n = 414), the PRS also significantly predicted epipodophyllotoxin-related SMN risk. The greatest improvements in risk prediction were for survivors who were exposed to epipodophyllotoxins (HR, 2.68; P < .01).
Screening and Follow-Up for Subsequent Neoplasms
Vigilant screening is important for childhood cancer survivors at risk.[120] Because of the relatively small size of the pediatric cancer survivor population and the prevalence and time to onset of therapy-related complications, undertaking clinical studies to assess the impact of screening recommendations on the morbidity and mortality associated with the late effect is not feasible.
Well-conducted studies of large populations of childhood cancer survivors have provided compelling evidence linking specific therapeutic exposures and late effects. This evidence has been used by several national and international cooperative groups (Scottish Collegiate Guidelines Network, Children's Cancer and Leukaemia Group, Children's Oncology Group [COG], DCOG) to develop consensus-based clinical practice guidelines to increase awareness and standardize the immediate care needs of medically vulnerable childhood cancer survivors.[121]
All pediatric cancer survivor health screening guidelines employ a hybrid approach that is both evidence-based (using established associations between therapeutic exposures and late effects to identify high-risk categories) and grounded in the collective clinical experience of experts (matching the magnitude of the risk with the intensity of the screening recommendations). The screening recommendations in these guidelines represent a statement of consensus from a panel of experts in the late effects of pediatric cancer treatment.[120,121]
The COG Guidelines for malignant SNs indicate that certain high-risk populations of childhood cancer survivors merit heightened surveillance because of predisposing host, behavioral, or therapeutic factors.[120]
References:
-
Mertens AC, Liu Q, Neglia JP, et al.: Cause-specific late mortality among 5-year survivors of childhood cancer: the Childhood Cancer Survivor Study. J Natl Cancer Inst 100 (19): 1368-79, 2008.
-
Friedman DL, Whitton J, Leisenring W, et al.: Subsequent neoplasms in 5-year survivors of childhood cancer: the Childhood Cancer Survivor Study. J Natl Cancer Inst 102 (14): 1083-95, 2010.
-
Turcotte LM, Whitton JA, Friedman DL, et al.: Risk of Subsequent Neoplasms During the Fifth and Sixth Decades of Life in the Childhood Cancer Survivor Study Cohort. J Clin Oncol 33 (31): 3568-75, 2015.
-
Turcotte LM, Liu Q, Yasui Y, et al.: Temporal Trends in Treatment and Subsequent Neoplasm Risk Among 5-Year Survivors of Childhood Cancer, 1970-2015. JAMA 317 (8): 814-824, 2017.
-
Stiller CA, Bunch KJ, Bayne AM, et al.: Subsequent cancers within 5 years from initial diagnosis of childhood cancer. Patterns and risks in the population of Great Britain. Pediatr Blood Cancer 70 (5): e30258, 2023.
-
Withrow DR, Anderson H, Armstrong GT, et al.: Pooled Analysis of Meningioma Risk Following Treatment for Childhood Cancer. JAMA Oncol 8 (12): 1756-1764, 2022.
-
Armstrong GT, Liu W, Leisenring W, et al.: Occurrence of multiple subsequent neoplasms in long-term survivors of childhood cancer: a report from the childhood cancer survivor study. J Clin Oncol 29 (22): 3056-64, 2011.
-
van Eggermond AM, Schaapveld M, Lugtenburg PJ, et al.: Risk of multiple primary malignancies following treatment of Hodgkin lymphoma. Blood 124 (3): 319-27; quiz 466, 2014.
-
Bowers DC, Moskowitz CS, Chou JF, et al.: Morbidity and Mortality Associated With Meningioma After Cranial Radiotherapy: A Report From the Childhood Cancer Survivor Study. J Clin Oncol 35 (14): 1570-1576, 2017.
-
Kok JL, Teepen JC, van Leeuwen FE, et al.: Risk of benign meningioma after childhood cancer in the DCOG-LATER cohort: contributions of radiation dose, exposed cranial volume, and age. Neuro Oncol 21 (3): 392-403, 2019.
-
Verbruggen LC, Kok JL, Teepen JC, et al.: Clinical characteristics of subsequent histologically confirmed meningiomas in long-term childhood cancer survivors: A Dutch LATER study. Eur J Cancer 150: 240-249, 2021.
-
Turcotte LM, Liu Q, Yasui Y, et al.: Chemotherapy and Risk of Subsequent Malignant Neoplasms in the Childhood Cancer Survivor Study Cohort. J Clin Oncol 37 (34): 3310-3319, 2019.
-
Teepen JC, van Leeuwen FE, Tissing WJ, et al.: Long-Term Risk of Subsequent Malignant Neoplasms After Treatment of Childhood Cancer in the DCOG LATER Study Cohort: Role of Chemotherapy. J Clin Oncol 35 (20): 2288-2298, 2017.
-
Wang Z, Wilson CL, Easton J, et al.: Genetic Risk for Subsequent Neoplasms Among Long-Term Survivors of Childhood Cancer. J Clin Oncol 36 (20): 2078-2087, 2018.
-
Allodji RS, Hawkins MM, Bright CJ, et al.: Risk of subsequent primary leukaemias among 69,460 five-year survivors of childhood cancer diagnosed from 1940 to 2008 in Europe: A cohort study within PanCareSurFup. Eur J Cancer 117: 71-83, 2019.
-
Allodji RS, Tucker MA, Hawkins MM, et al.: Role of radiotherapy and chemotherapy in the risk of leukemia after childhood cancer: An international pooled analysis. Int J Cancer 148 (9): 2079-2089, 2021.
-
Bhatia S, Yasui Y, Robison LL, et al.: High risk of subsequent neoplasms continues with extended follow-up of childhood Hodgkin's disease: report from the Late Effects Study Group. J Clin Oncol 21 (23): 4386-94, 2003.
-
Nottage K, Lanctot J, Li Z, et al.: Long-term risk for subsequent leukemia after treatment for childhood cancer: a report from the Childhood Cancer Survivor Study. Blood 117 (23): 6315-8, 2011.
-
Khoury JD, Solary E, Abla O, et al.: The 5th edition of the World Health Organization Classification of Haematolymphoid Tumours: Myeloid and Histiocytic/Dendritic Neoplasms. Leukemia 36 (7): 1703-1719, 2022.
-
Thirman MJ, Larson RA: Therapy-related myeloid leukemia. Hematol Oncol Clin North Am 10 (2): 293-320, 1996.
-
Pedersen-Bjergaard J, Philip P: Balanced translocations involving chromosome bands 11q23 and 21q22 are highly characteristic of myelodysplasia and leukemia following therapy with cytostatic agents targeting at DNA-topoisomerase II. Blood 78 (4): 1147-8, 1991.
-
Kok JL, Teepen JC, van der Pal HJ, et al.: Incidence of and Risk Factors for Histologically Confirmed Solid Benign Tumors Among Long-term Survivors of Childhood Cancer. JAMA Oncol 5 (5): 671-680, 2019.
-
Inskip PD, Sigurdson AJ, Veiga L, et al.: Radiation-Related New Primary Solid Cancers in the Childhood Cancer Survivor Study: Comparative Radiation Dose Response and Modification of Treatment Effects. Int J Radiat Oncol Biol Phys 94 (4): 800-7, 2016.
-
Ehrhardt MJ, Howell CR, Hale K, et al.: Subsequent Breast Cancer in Female Childhood Cancer Survivors in the St Jude Lifetime Cohort Study (SJLIFE). J Clin Oncol 37 (19): 1647-1656, 2019.
-
Taylor AJ, Little MP, Winter DL, et al.: Population-based risks of CNS tumors in survivors of childhood cancer: the British Childhood Cancer Survivor Study. J Clin Oncol 28 (36): 5287-93, 2010.
-
Henderson TO, Whitton J, Stovall M, et al.: Secondary sarcomas in childhood cancer survivors: a report from the Childhood Cancer Survivor Study. J Natl Cancer Inst 99 (4): 300-8, 2007.
-
Veiga LH, Holmberg E, Anderson H, et al.: Thyroid Cancer after Childhood Exposure to External Radiation: An Updated Pooled Analysis of 12 Studies. Radiat Res 185 (5): 473-84, 2016.
-
Reulen RC, Frobisher C, Winter DL, et al.: Long-term risks of subsequent primary neoplasms among survivors of childhood cancer. JAMA 305 (22): 2311-9, 2011.
-
Teepen JC, Kok JL, Kremer LC, et al.: Long-Term Risk of Skin Cancer Among Childhood Cancer Survivors: A DCOG-LATER Cohort Study. J Natl Cancer Inst 111 (8): 845-853, 2019.
-
Majhail NS, Brazauskas R, Rizzo JD, et al.: Secondary solid cancers after allogeneic hematopoietic cell transplantation using busulfan-cyclophosphamide conditioning. Blood 117 (1): 316-22, 2011.
-
Baker KS, Leisenring WM, Goodman PJ, et al.: Total body irradiation dose and risk of subsequent neoplasms following allogeneic hematopoietic cell transplantation. Blood 133 (26): 2790-2799, 2019.
-
Veiga LH, Curtis RE, Morton LM, et al.: Association of Breast Cancer Risk After Childhood Cancer With Radiation Dose to the Breast and Anthracycline Use: A Report From the Childhood Cancer Survivor Study. JAMA Pediatr 173 (12): 1171-1179, 2019.
-
Kenney LB, Yasui Y, Inskip PD, et al.: Breast cancer after childhood cancer: a report from the Childhood Cancer Survivor Study. Ann Intern Med 141 (8): 590-7, 2004.
-
Moskowitz CS, Chou JF, Wolden SL, et al.: Breast cancer after chest radiation therapy for childhood cancer. J Clin Oncol 32 (21): 2217-23, 2014.
-
Henderson TO, Liu Q, Turcotte LM, et al.: Association of Changes in Cancer Therapy Over 3 Decades With Risk of Subsequent Breast Cancer Among Female Childhood Cancer Survivors: A Report From the Childhood Cancer Survivor Study (CCSS). JAMA Oncol : , 2022.
-
Schaapveld M, Aleman BM, van Eggermond AM, et al.: Second Cancer Risk Up to 40 Years after Treatment for Hodgkin's Lymphoma. N Engl J Med 373 (26): 2499-511, 2015.
-
Travis LB, Hill D, Dores GM, et al.: Cumulative absolute breast cancer risk for young women treated for Hodgkin lymphoma. J Natl Cancer Inst 97 (19): 1428-37, 2005.
-
O'Brien MM, Donaldson SS, Balise RR, et al.: Second malignant neoplasms in survivors of pediatric Hodgkin's lymphoma treated with low-dose radiation and chemotherapy. J Clin Oncol 28 (7): 1232-9, 2010.
-
Inskip PD, Robison LL, Stovall M, et al.: Radiation dose and breast cancer risk in the childhood cancer survivor study. J Clin Oncol 27 (24): 3901-7, 2009.
-
Travis LB, Hill DA, Dores GM, et al.: Breast cancer following radiotherapy and chemotherapy among young women with Hodgkin disease. JAMA 290 (4): 465-75, 2003.
-
van Leeuwen FE, Klokman WJ, Stovall M, et al.: Roles of radiation dose, chemotherapy, and hormonal factors in breast cancer following Hodgkin's disease. J Natl Cancer Inst 95 (13): 971-80, 2003.
-
Lange JM, Takashima JR, Peterson SM, et al.: Breast cancer in female survivors of Wilms tumor: a report from the national Wilms tumor late effects study. Cancer 120 (23): 3722-30, 2014.
-
Wang Y, Ronckers CM, van Leeuwen FE, et al.: Subsequent female breast cancer risk associated with anthracycline chemotherapy for childhood cancer. Nat Med 29 (9): 2268-2277, 2023.
-
Henderson TO, Moskowitz CS, Chou JF, et al.: Breast Cancer Risk in Childhood Cancer Survivors Without a History of Chest Radiotherapy: A Report From the Childhood Cancer Survivor Study. J Clin Oncol 34 (9): 910-8, 2016.
-
Eisenberg ER, Weiss A, Prakash I, et al.: Surgical Management and Contralateral Breast Cancer Risk in Women with History of Radiation Therapy for Hodgkin Lymphoma: Results from a Population-Based Cohort. Ann Surg Oncol 29 (11): 6673-6680, 2022.
-
Moskowitz CS, Ronckers CM, Chou JF, et al.: Development and Validation of a Breast Cancer Risk Prediction Model for Childhood Cancer Survivors Treated With Chest Radiation: A Report From the Childhood Cancer Survivor Study and the Dutch Hodgkin Late Effects and LATER Cohorts. J Clin Oncol 39 (27): 3012-3021, 2021.
-
Dores GM, Anderson WF, Beane Freeman LE, et al.: Risk of breast cancer according to clinicopathologic features among long-term survivors of Hodgkin's lymphoma treated with radiotherapy. Br J Cancer 103 (7): 1081-4, 2010.
-
Horst KC, Hancock SL, Ognibene G, et al.: Histologic subtypes of breast cancer following radiotherapy for Hodgkin lymphoma. Ann Oncol 25 (4): 848-51, 2014.
-
Demoor-Goldschmidt C, Supiot S, Mahé MA, et al.: Clinical and histological features of second breast cancers following radiotherapy for childhood and young adult malignancy. Br J Radiol 91 (1086): 20170824, 2018.
-
Castiglioni F, Terenziani M, Carcangiu ML, et al.: Radiation effects on development of HER2-positive breast carcinomas. Clin Cancer Res 13 (1): 46-51, 2007.
-
Gaffney DK, Hemmersmeier J, Holden J, et al.: Breast cancer after mantle irradiation for Hodgkin's disease: correlation of clinical, pathologic, and molecular features including loss of heterozygosity at BRCA1 and BRCA2. Int J Radiat Oncol Biol Phys 49 (2): 539-46, 2001.
-
Wong SM, Ajjamada L, Weiss AC, et al.: Clinicopathologic features of breast cancers diagnosed in women treated with prior radiation therapy for Hodgkin lymphoma: Results from a population-based cohort. Cancer 128 (7): 1365-1372, 2022.
-
Moskowitz CS, Chou JF, Neglia JP, et al.: Mortality After Breast Cancer Among Survivors of Childhood Cancer: A Report From the Childhood Cancer Survivor Study. J Clin Oncol 37 (24): 2120-2130, 2019.
-
Yeh JM, Lowry KP, Schechter CB, et al.: Clinical Benefits, Harms, and Cost-Effectiveness of Breast Cancer Screening for Survivors of Childhood Cancer Treated With Chest Radiation : A Comparative Modeling Study. Ann Intern Med 173 (5): 331-341, 2020.
-
van Santen HM, Tytgat GA, van de Wetering MD, et al.: Differentiated thyroid carcinoma after 131I-MIBG treatment for neuroblastoma during childhood: description of the first two cases. Thyroid 22 (6): 643-6, 2012.
-
Vivanco M, Dalle JH, Alberti C, et al.: Malignant and benign thyroid nodules after total body irradiation preceding hematopoietic cell transplantation during childhood. Eur J Endocrinol 167 (2): 225-33, 2012.
-
Michaelson EM, Chen YH, Silver B, et al.: Thyroid malignancies in survivors of Hodgkin lymphoma. Int J Radiat Oncol Biol Phys 88 (3): 636-41, 2014.
-
Sigurdson AJ, Ronckers CM, Mertens AC, et al.: Primary thyroid cancer after a first tumour in childhood (the Childhood Cancer Survivor Study): a nested case-control study. Lancet 365 (9476): 2014-23, 2005 Jun 11-17.
-
Clement SC, Lebbink CA, Klein Hesselink MS, et al.: Presentation and outcome of subsequent thyroid cancer among childhood cancer survivors compared to sporadic thyroid cancer: a matched national study. Eur J Endocrinol 183 (2): 169-180, 2020.
-
Neglia JP, Robison LL, Stovall M, et al.: New primary neoplasms of the central nervous system in survivors of childhood cancer: a report from the Childhood Cancer Survivor Study. J Natl Cancer Inst 98 (21): 1528-37, 2006.
-
Hijiya N, Hudson MM, Lensing S, et al.: Cumulative incidence of secondary neoplasms as a first event after childhood acute lymphoblastic leukemia. JAMA 297 (11): 1207-15, 2007.
-
Faraci M, Morana G, Bagnasco F, et al.: Magnetic resonance imaging in childhood leukemia survivors treated with cranial radiotherapy: a cross sectional, single center study. Pediatr Blood Cancer 57 (2): 240-6, 2011.
-
Vinchon M, Leblond P, Caron S, et al.: Radiation-induced tumors in children irradiated for brain tumor: a longitudinal study. Childs Nerv Syst 27 (3): 445-53, 2011.
-
Koike T, Yanagimachi N, Ishiguro H, et al.: High incidence of radiation-induced cavernous hemangioma in long-term survivors who underwent hematopoietic stem cell transplantation with radiation therapy during childhood or adolescence. Biol Blood Marrow Transplant 18 (7): 1090-8, 2012.
-
Bowers DC, Verbruggen LC, Kremer LCM, et al.: Surveillance for subsequent neoplasms of the CNS for childhood, adolescent, and young adult cancer survivors: a systematic review and recommendations from the International Late Effects of Childhood Cancer Guideline Harmonization Group. Lancet Oncol 22 (5): e196-e206, 2021.
-
Baliga S, Gallotto S, Bajaj B, et al.: Decade-long disease, secondary malignancy, and brainstem injury outcomes in pediatric and young adult medulloblastoma patients treated with proton radiotherapy. Neuro Oncol 24 (6): 1010-1019, 2022.
-
Wong JR, Morton LM, Tucker MA, et al.: Risk of subsequent malignant neoplasms in long-term hereditary retinoblastoma survivors after chemotherapy and radiotherapy. J Clin Oncol 32 (29): 3284-90, 2014.
-
Fidler MM, Reulen RC, Winter DL, et al.: Risk of Subsequent Bone Cancers Among 69 460 Five-Year Survivors of Childhood and Adolescent Cancer in Europe. J Natl Cancer Inst 110 (2): , 2018.
-
Bright CJ, Hawkins MM, Winter DL, et al.: Risk of Soft-Tissue Sarcoma Among 69 460 Five-Year Survivors of Childhood Cancer in Europe. J Natl Cancer Inst 110 (6): 649-660, 2018.
-
Chaussade A, Millot G, Wells C, et al.: Correlation between RB1germline mutations and second primary malignancies in hereditary retinoblastoma patients treated with external beam radiotherapy. Eur J Med Genet 62 (3): 217-223, 2019.
-
Kube SJ, Blattmann C, Bielack SS, et al.: Secondary malignant neoplasms after bone and soft tissue sarcomas in children, adolescents, and young adults. Cancer 128 (9): 1787-1800, 2022.
-
Schwartz B, Benadjaoud MA, Cléro E, et al.: Risk of second bone sarcoma following childhood cancer: role of radiation therapy treatment. Radiat Environ Biophys 53 (2): 381-90, 2014.
-
Bielack SS, Blattmann C, Hassenpflug W, et al.: Osteosarcoma Arising After Ewing Sarcoma or Vice Versa: A Report of 20 Affected Patients from the Cooperative Osteosarcoma Study Group (COSS). Anticancer Res 43 (11): 4975-4981, 2023.
-
Henderson TO, Rajaraman P, Stovall M, et al.: Risk factors associated with secondary sarcomas in childhood cancer survivors: a report from the childhood cancer survivor study. Int J Radiat Oncol Biol Phys 84 (1): 224-30, 2012.
-
Reulen RC, Winter DL, Diallo I, et al.: Risk Factors for Primary Bone Cancer After Childhood Cancer: A PanCare Childhood and Adolescent Cancer Survivor Care and Follow-Up Studies Nested Case-Control Study. J Clin Oncol 41 (21): 3735-3746, 2023.
-
MacCarthy A, Bayne AM, Brownbill PA, et al.: Second and subsequent tumours among 1927 retinoblastoma patients diagnosed in Britain 1951-2004. Br J Cancer 108 (12): 2455-63, 2013.
-
Temming P, Arendt M, Viehmann A, et al.: Incidence of second cancers after radiotherapy and systemic chemotherapy in heritable retinoblastoma survivors: A report from the German reference center. Pediatr Blood Cancer 64 (1): 71-80, 2017.
-
Kleinerman RA, Schonfeld SJ, Sigel BS, et al.: Bone and Soft-Tissue Sarcoma Risk in Long-Term Survivors of Hereditary Retinoblastoma Treated With Radiation. J Clin Oncol 37 (35): 3436-3445, 2019.
-
Thorsness SL, Freites-Martinez A, Marchetti MA, et al.: Nonmelanoma Skin Cancer in Childhood and Young Adult Cancer Survivors Previously Treated With Radiotherapy. J Natl Compr Canc Netw 17 (3): 237-243, 2019.
-
Geller AC, Coroiu A, Keske RR, et al.: Advancing Survivors Knowledge (ASK Study) of Skin Cancer Surveillance After Childhood Cancer: A Randomized Controlled Trial in the Childhood Cancer Survivor Study. J Clin Oncol 41 (12): 2269-2280, 2023.
-
Watt TC, Inskip PD, Stratton K, et al.: Radiation-related risk of basal cell carcinoma: a report from the Childhood Cancer Survivor Study. J Natl Cancer Inst 104 (16): 1240-50, 2012.
-
Braam KI, Overbeek A, Kaspers GJ, et al.: Malignant melanoma as second malignant neoplasm in long-term childhood cancer survivors: a systematic review. Pediatr Blood Cancer 58 (5): 665-74, 2012.
-
Pappo AS, Armstrong GT, Liu W, et al.: Melanoma as a subsequent neoplasm in adult survivors of childhood cancer: a report from the childhood cancer survivor study. Pediatr Blood Cancer 60 (3): 461-6, 2013.
-
Kleinerman RA, Schonfeld SJ, Abramson DH, et al.: Increased Risk of Skin Cancer in 1,851 Long-Term Retinoblastoma Survivors. J Invest Dermatol 141 (12): 2849-2857.e3, 2021.
-
Ghosh T, Chen Y, Dietz AC, et al.: Lung Cancer as a Subsequent Malignant Neoplasm in Survivors of Childhood Cancer. Cancer Epidemiol Biomarkers Prev 30 (12): 2235-2243, 2021.
-
van Leeuwen FE, Klokman WJ, Stovall M, et al.: Roles of radiotherapy and smoking in lung cancer following Hodgkin's disease. J Natl Cancer Inst 87 (20): 1530-7, 1995.
-
Henderson TO, Oeffinger KC, Whitton J, et al.: Secondary gastrointestinal cancer in childhood cancer survivors: a cohort study. Ann Intern Med 156 (11): 757-66, W-260, 2012.
-
Nottage K, McFarlane J, Krasin MJ, et al.: Secondary colorectal carcinoma after childhood cancer. J Clin Oncol 30 (20): 2552-8, 2012.
-
Heymer EJ, Jóźwiak K, Kremer LC, et al.: Cumulative Absolute Risk of Subsequent Colorectal Cancer After Abdominopelvic Radiotherapy Among Childhood Cancer Survivors: A PanCareSurFup Study. J Clin Oncol 42 (3): 336-347, 2024.
-
Tukenova M, Diallo I, Anderson H, et al.: Second malignant neoplasms in digestive organs after childhood cancer: a cohort-nested case-control study. Int J Radiat Oncol Biol Phys 82 (3): e383-90, 2012.
-
Daly PE, Samiee S, Cino M, et al.: High prevalence of adenomatous colorectal polyps in young cancer survivors treated with abdominal radiation therapy: results of a prospective trial. Gut 66 (10): 1797-1801, 2017.
-
Teepen JC, Kok JL, van Leeuwen FE, et al.: Colorectal Adenomas and Cancers After Childhood Cancer Treatment: A DCOG-LATER Record Linkage Study. J Natl Cancer Inst 110 (7): 758-767, 2018.
-
Reulen RC, Wong KF, Bright CJ, et al.: Risk of digestive cancers in a cohort of 69 460 five-year survivors of childhood cancer in Europe: the PanCareSurFup study. Gut : , 2020.
-
Geurts YM, Shakir R, Ntentas G, et al.: Association of Radiation and Procarbazine Dose With Risk of Colorectal Cancer Among Survivors of Hodgkin Lymphoma. JAMA Oncol 9 (4): 481-489, 2023.
-
Sunguc C, Hawkins MM, Winter DL, et al.: Risk of subsequent primary oral cancer in a cohort of 69,460 5-year survivors of childhood and adolescent cancer in Europe: the PanCareSurFup study. Br J Cancer 128 (1): 80-90, 2023.
-
Liu JJ, De Vivo I, Wu CY, et al.: Subsequent primary urogenital cancers among childhood and adolescent cancer survivors in the United States. Urol Oncol 40 (2): 65.e11-65.e18, 2022.
-
Wilson CL, Ness KK, Neglia JP, et al.: Renal carcinoma after childhood cancer: a report from the childhood cancer survivor study. J Natl Cancer Inst 105 (7): 504-8, 2013.
-
de Vathaire F, Scwhartz B, El-Fayech C, et al.: Risk of a Second Kidney Carcinoma Following Childhood Cancer: Role of Chemotherapy and Radiation Dose to Kidneys. J Urol 194 (5): 1390-5, 2015.
-
Hedgepeth RC, Zhou M, Ross J: Rapid development of metastatic Xp11 translocation renal cell carcinoma in a girl treated for neuroblastoma. J Pediatr Hematol Oncol 31 (8): 602-4, 2009.
-
Argani P, Laé M, Ballard ET, et al.: Translocation carcinomas of the kidney after chemotherapy in childhood. J Clin Oncol 24 (10): 1529-34, 2006.
-
Ma J, Pan C, Yin M: Translocation Renal Cell Carcinoma in a Child Previously Treated for Infantile Fibrosarcoma. Pediatr Dev Pathol 21 (4): 418-422, 2018 Jul-Aug.
-
Fleitz JM, Wootton-Gorges SL, Wyatt-Ashmead J, et al.: Renal cell carcinoma in long-term survivors of advanced stage neuroblastoma in early childhood. Pediatr Radiol 33 (8): 540-5, 2003.
-
Henderson TO, Fowler BW, Hamann HA, et al.: Subsequent malignant neoplasms in the Childhood Cancer Survivor Study: Occurrence of cancer types in which human papillomavirus is an established etiologic risk factor. Cancer 128 (2): 373-382, 2022.
-
Ou JY, Bennion N, Parker K, et al.: Risk Factors and Trends for HPV-Associated Subsequent Malignant Neoplasms among Adolescent and Young Adult Cancer Survivors. Cancer Epidemiol Biomarkers Prev 32 (5): 625-633, 2023.
-
Brown AL, Arroyo VM, Agrusa JE, et al.: Survival disparities for second primary malignancies diagnosed among childhood cancer survivors: A population-based assessment. Cancer 125 (20): 3623-3630, 2019.
-
Archer NM, Amorim RP, Naves R, et al.: An Increased Risk of Second Malignant Neoplasms After Rhabdomyosarcoma: Population-Based Evidence for a Cancer Predisposition Syndrome? Pediatr Blood Cancer 63 (2): 196-201, 2016.
-
Wang X, Sun CL, Hageman L, et al.: Clinical and Genetic Risk Prediction of Subsequent CNS Tumors in Survivors of Childhood Cancer: A Report From the COG ALTE03N1 Study. J Clin Oncol 35 (32): 3688-3696, 2017.
-
Waespe N, Belle FN, Redmond S, et al.: Cancer predisposition syndromes as a risk factor for early second primary neoplasms after childhood cancer - A national cohort study. Eur J Cancer 145: 71-80, 2021.
-
Andersson A, Enblad G, Tavelin B, et al.: Family history of cancer as a risk factor for second malignancies after Hodgkin's lymphoma. Br J Cancer 98 (5): 1001-5, 2008.
-
Hisada M, Garber JE, Fung CY, et al.: Multiple primary cancers in families with Li-Fraumeni syndrome. J Natl Cancer Inst 90 (8): 606-11, 1998.
-
de Andrade KC, Khincha PP, Hatton JN, et al.: Cancer incidence, patterns, and genotype-phenotype associations in individuals with pathogenic or likely pathogenic germline TP53 variants: an observational cohort study. Lancet Oncol 22 (12): 1787-1798, 2021.
-
Bhatia S, Chen Y, Wong FL, et al.: Subsequent Neoplasms After a Primary Tumor in Individuals With Neurofibromatosis Type 1. J Clin Oncol 37 (32): 3050-3058, 2019.
-
de Blank P, Li N, Fisher MJ, et al.: Late morbidity and mortality in adult survivors of childhood glioma with neurofibromatosis type 1: report from the Childhood Cancer Survivor Study. Genet Med 22 (11): 1794-1802, 2020.
-
Strahm B, Malkin D: Hereditary cancer predisposition in children: genetic basis and clinical implications. Int J Cancer 119 (9): 2001-6, 2006.
-
Cullinan N, Schiller I, Di Giuseppe G, et al.: Utility of a Cancer Predisposition Screening Tool for Predicting Subsequent Malignant Neoplasms in Childhood Cancer Survivors. J Clin Oncol 39 (29): 3207-3216, 2021.
-
Goudie C, Witkowski L, Cullinan N, et al.: Performance of the McGill Interactive Pediatric OncoGenetic Guidelines for Identifying Cancer Predisposition Syndromes. JAMA Oncol 7 (12): 1806-1814, 2021.
-
Qin N, Wang Z, Liu Q, et al.: Pathogenic Germline Mutations in DNA Repair Genes in Combination With Cancer Treatment Exposures and Risk of Subsequent Neoplasms Among Long-Term Survivors of Childhood Cancer. J Clin Oncol 38 (24): 2728-2740, 2020.
-
Collins A, Harrington V: Repair of oxidative DNA damage: assessing its contribution to cancer prevention. Mutagenesis 17 (6): 489-93, 2002.
-
Im C, Sharafeldin N, Yuan Y, et al.: Polygenic Risk and Chemotherapy-Related Subsequent Malignancies in Childhood Cancer Survivors: A Childhood Cancer Survivor Study and St Jude Lifetime Cohort Study Report. J Clin Oncol 41 (27): 4381-4393, 2023.
-
Landier W, Bhatia S, Eshelman DA, et al.: Development of risk-based guidelines for pediatric cancer survivors: the Children's Oncology Group Long-Term Follow-Up Guidelines from the Children's Oncology Group Late Effects Committee and Nursing Discipline. J Clin Oncol 22 (24): 4979-90, 2004.
-
Kremer LC, Mulder RL, Oeffinger KC, et al.: A worldwide collaboration to harmonize guidelines for the long-term follow-up of childhood and young adult cancer survivors: a report from the International Late Effects of Childhood Cancer Guideline Harmonization Group. Pediatr Blood Cancer 60 (4): 543-9, 2013.
-
Mulder RL, Hudson MM, Bhatia S, et al.: Updated Breast Cancer Surveillance Recommendations for Female Survivors of Childhood, Adolescent, and Young Adult Cancer From the International Guideline Harmonization Group. J Clin Oncol 38 (35): 4194-4207, 2020.
-
Kriege M, Brekelmans CT, Boetes C, et al.: Efficacy of MRI and mammography for breast-cancer screening in women with a familial or genetic predisposition. N Engl J Med 351 (5): 427-37, 2004.
-
Leach MO, Boggis CR, Dixon AK, et al.: Screening with magnetic resonance imaging and mammography of a UK population at high familial risk of breast cancer: a prospective multicentre cohort study (MARIBS). Lancet 365 (9473): 1769-78, 2005 May 21-27.
-
Tieu MT, Cigsar C, Ahmed S, et al.: Breast cancer detection among young survivors of pediatric Hodgkin lymphoma with screening magnetic resonance imaging. Cancer 120 (16): 2507-13, 2014.
-
Saslow D, Boetes C, Burke W, et al.: American Cancer Society guidelines for breast screening with MRI as an adjunct to mammography. CA Cancer J Clin 57 (2): 75-89, 2007 Mar-Apr.
-
Berrington de Gonzalez A, Berg CD, Visvanathan K, et al.: Estimated risk of radiation-induced breast cancer from mammographic screening for young BRCA mutation carriers. J Natl Cancer Inst 101 (3): 205-9, 2009.
-
Young KC, Burch A, Oduko JM: Radiation doses received in the UK Breast Screening Programme in 2001 and 2002. Br J Radiol 78 (927): 207-18, 2005.
-
Spelic DC: Trends in Mammography Dose and Image Quality 1974-2005. Silver Spring, Md: U.S. Food and Drug Administration, 2006. Available online. Last accessed August 21, 2023.
-
Clement SC, Kremer LCM, Verburg FA, et al.: Balancing the benefits and harms of thyroid cancer surveillance in survivors of Childhood, adolescent and young adult cancer: Recommendations from the international Late Effects of Childhood Cancer Guideline Harmonization Group in collaboration with the PanCareSurFup Consortium. Cancer Treat Rev 63: 28-39, 2018.
Late Effects of the Cardiovascular System
Cardiovascular disease, after recurrence of the original cancer and development of second primary cancers, has been reported to be the leading cause of premature mortality among long-term childhood cancer survivors.[1,2,3]
Evidence (excess risk of premature cardiovascular mortality):
- In the North American Childhood Cancer Survivor Study (CCSS), more than 34,000 5-year survivors of childhood cancer were diagnosed and treated from 1970 to 1999.[1]
- Participants followed for 15 years had a standardized mortality ratio (SMR) of 11.7 (95% confidence interval [CI], 9.4–14.4) for cardiac mortality.
- Late cardiac mortality in children who were treated more recently (i.e., in the 1990s) appears to have decreased (e.g., the cumulative incidence was 0.5% in 1970–1974 vs. 0.1% in 1990–1994).
- In the CCSS, outcomes for patients diagnosed and treated in adolescence and early adulthood (aged >15 to <21 years) were compared with survivors of similar cancers diagnosed in childhood (aged <15 years) and the general population.[4]
- Childhood cancer survivors (n = 5,804) had a greater SMR than early-adolescent and young adult survivors (n = 5,804) for cardiac deaths beginning 20 years after diagnosis (SMR, 5.4; 95% CI, 3.7–7.8 vs. SMR, 1.8; 95% CI, 0.7–4.3).
- When compared with siblings of the same age, survivors of childhood cancer (n = 4,082) had a higher risk of developing grades 3 to 5 chronic cardiac conditions than early adolescents and young adults (n = 4,082) (hazard ratio [HR], 5.6 [4.5–7.1] vs. 4.3 [3.5–5.4]).
- Cardiac disease becomes increasingly important as survivors of childhood cancer reach mature adulthood. This finding was observed in the population-based British Childhood Cancer Survivor Study, comprised of 34,489 5-year survivors of childhood cancer diagnosed from 1940 to 2006.[2,5]
- In survivors of childhood cancer aged 60 years and older, circulatory disease overtakes subsequent primary neoplasms as the leading cause of excess mortality (37% of the excess number of deaths observed were caused by circulatory conditions compared with 31% of excess number of deaths caused by subsequent primary neoplasms).[2]
- The risk of both overall cardiac and cardiomyopathy/heart failure mortality was greatest among those diagnosed between 1980 and 1989. Survivors who were diagnosed from 1980 to 1989 had 28.9 times the excess number of cardiac deaths as did survivors who were diagnosed either before 1970 or from 1990 to the present.[5]
The specific late effects covered in this section include the following:
- Cardiomyopathy/heart failure.
- Ischemic heart disease.
- Pericardial heart disease.
- Valve disease.
- Conduction disorders.
- Cerebrovascular disease.
- Venous thromboembolism.
This section will also briefly discuss the influence of related conditions such as hypertension, dyslipidemia, and diabetes. However, this section will not provide a detailed review of those conditions as a consequence of childhood cancer treatment. A comprehensive review of long-term cardiovascular toxicity in childhood and young adult survivors of cancer has been published.[6]
Cardiovascular Outcomes
- Numerous studies focus on cardiac events among childhood cancer survivors. Very large cohort studies exist, many with several decades of follow-up, that are either hospital based,[7,8,9] clinical trial based,[10,11] or population based.[2,3,5]
- Notably, the average age of these populations is still relatively young (early or mid-adulthood). Consequently, the reported risk of serious cardiovascular outcomes is very high relative to the age-matched general population, whereas the absolute risk often remains low, limiting the power of many studies.
- Among the large studies featuring thousands of survivors, the main limitation has been inadequate ability to clinically ascertain late cardiovascular complications, with a greater reliance on either administrative records (e.g., death registries) and/or self-report or proxy-report.
- While each study design has some inherent biases, the overall literature, based on a combination of self-reported outcomes, clinical ascertainment, and administrative data sources, is robust. These studies conclude that certain cancer-related exposures are associated with a significantly greater risk of cardiovascular morbidity and mortality in survivors.
- Although late effects research often lags behind changes in contemporary therapy, many therapies linked to cardiovascular late effects remain in common use today. However, restricted doses of these therapies are often given to patients with biologically favorable disease presentations. In addition, dexrazoxane cardioprotection is also widely used for patients exposed to these therapies.[12,13,14]
- Ongoing research is important to ensure that use of newer targeted agents does not result in unexpected cardiovascular effects.[15]
Evidence (selected cohort studies describing cardiovascular outcomes):
- A case-control study of the PanCareSurFup and ProCardio cohort evaluated treatment-related risk factors for heart failure in survivors of childhood cancer (5 or more years) diagnosed between 1940 and 2009.[16]
- The 50-year cumulative incidence of heart failure among the entire cohort was 2% (95% CI, 1.7%–2.2%).
- A subcohort study evaluated 500 cases with symptomatic heart failure and 500 controls without heart failure (matched for sex, age, calendar year of first diagnosis, and length of follow-up) for whom detailed treatment exposures were available. The risk of heart failure was increased more than fivefold (odds ratio [OR], 5.5; 95% CI, 2.5–12.3) among survivors who received a mean heart radiation therapy dose of 5 Gy to 15 Gy or less, compared with those who did not receive heart radiation therapy. The risk increased with a larger volume of radiation exposing the heart.
- Heart failure risk increased in a linear fashion with higher mean heart radiation therapy doses.
- Survivors who received a cumulative anthracycline dose of more than 100 mg/m2 had a substantially increased risk of heart failure (OR, 5.8; 95% CI, 2.9–11.3 for 100 to <250 mg/m2 and OR, 21.2; 95% CI, 11.4–39.2 for >250 mg/m2).
- An anthracycline dose of less than 100 mg/m2 was not a significant risk factor for heart failure.
- CCSS investigators reported on major cardiac events among participants diagnosed with childhood cancer between 1970 and 1999.[17]
- In this update, the 20-year cumulative incidence of heart failure and coronary artery disease for patients treated in the 1990s declined over the decades to 0.54% and 0.19%, respectively, but was significant only for coronary artery disease.
- The risk of coronary artery disease was significantly decreased from the 1970s, 1980s, and 1990s (0.38%, 0.24%, and 0.19%, respectively; HR, 0.65) and was attributed to historical reductions in exposure to cardiac radiation, particularly among survivors of Hodgkin lymphoma.
- For patients treated in the 1990s, the 20-year cumulative incidence was 0.05% for valvular disease, 0.03% for pericardial disease, and 0.13% for arrhythmias. These numbers did not change over the eras (1970–1990).
- In the CCSS, data from 24,214 5-year survivors diagnosed between 1970 and 1999 were used to assess the impacts of radiation therapy dose and exposed cardiac volume, select chemotherapeutic agents, and age at exposure on the risk of late-onset cardiac disease.[18]
- The cumulative incidence of cardiac disease (any cardiac disease, coronary artery disease, and heart failure) 30 years from diagnosis was 4.8%. Male survivors were more likely to develop coronary artery disease and less likely to develop heart failure than were female survivors. Non-Hispanic Black survivors were more likely to develop any cardiac disease than were non-Hispanic White survivors.
- Low-to-moderate radiation therapy doses (5.0–19.9 Gy) to large cardiac volumes (>50% of the heart) were associated with a 1.6-fold increased risk of cardiac disease compared with survivors who did not have any cardiac radiation therapy exposure.
- High doses (>20 Gy) to small cardiac volumes (0.1%–29.9%) were associated with an elevated rate of cardiac disease compared with unexposed survivors.
- A dose-response relationship was observed between anthracycline exposure and heart failure, with younger children (<13 years) at the greatest risk of heart failure after comparable dosing.
- Another CCSS study evaluated radiation dose–response relationships for cardiac substructures and cardiac outcomes among 25,481 5-year survivors treated between 1970 to 1999. Of these survivors, 48.2% were exposed to radiation therapy.[19]
- At a median age of 30 years, the cumulative incidence (35 years from diagnosis) was 3.9% (95% CI, 3.4%–4.3%) for coronary artery disease, 3.8% (95% CI, 3.4%–4.2%) for heart failure, 1.2% (95% CI, 1.0%–1.5%) for valvular disease, and 1.4% (95% CI, 1.1%–1.96%) for arrythmia.
- Mean doses of 5 to 9.9 Gy to the whole heart did not increase the risk of any cardiac disease, whereas mean doses of 5 to 9.9 Gy to the right coronary artery (relative risk [RR], 2.6; 95% CI, 1.6–4.1) and left ventricle (RR, 2.2; 95% CI, 1.3–3.7) increased the risk of coronary artery disease.
- Mean doses of 5 to 9.9 Gy to the tricuspid valve (RR, 5.5; 95% CI, 2.0–15.1) and right ventricle (RR, 8.4; 95% CI, 3.7–19.0) increased the risk of valvular disease.
- Statistical modeling suggested that any radiation to the cardiac substructures elevate the risk of cardiac diseases, and there is no threshold dose that can mitigate this risk.
- A cross-sectional CCSS study included 571 adult-aged survivors of childhood cancer (median age, 37.7 years; 28.5 years from cancer diagnosis). The study compared rates of underdiagnosis and undertreatment of modifiable risk factors among survivors and respondents to the National Health and Nutrition Examination Survey (NHNES).[20]
- Rates of cardiovascular disease risk factor underdiagnosis were similar (27.1% of survivors vs. 26.1% of participants from the NHNES; P = .73). However, survivors were more likely to be undertreated (21.0% versus 13.9%, P = .007; OR, 1.8; 95% CI, 1.2–2.7).
- Hypertension (18.9%) and dyslipidemia (16.3%) represented the most underdiagnosed and undertreated risk factors.
- Men and survivors who were overweight or had obesity were more likely to be underdiagnosed and undertreated.
- These adult-aged childhood cancer survivors were nearly twice as likely to be undertreated for these conditions.
- Dyslipidemia is more common and is an independent predictor of cardiovascular disease among survivors, compared with controls. Specific lipid abnormalities and the risk of atherosclerotic cardiovascular disease were investigated in 4,115 childhood cancer survivors from the St. Jude Life (SJLIFE) cohort.[21]
- Of this cohort, 3,406 survivors did not have a previous dyslipidemia diagnosis.
- Low HDL cholesterol (HR, 2.9) and elevated triglycerides (HR, 3.1) were associated with an increased risk of myocardial infarction.
- Diagnosis of high LDL cholesterol (HR, 2.2), high non-HDL cholesterol (HR, 2.2), low HDL cholesterol (HR, 3.9), and elevated triglycerides (HR, 3.8) were associated with an increased risk of cardiomyopathy.
- A Dutch CCSS-LATER study evaluated the prevalence of and risk factors for hypertension in childhood cancer survivors (median age, 32.5 years; median follow-up, 25.5 years) who were treated with potentially nephrotoxic therapies.[22]
- Survivors (16.3%) and controls (18.2%) had comparable rates of hypertension. Undiagnosed hypertension was prevalent in 12% of survivors and 17.8% of controls.
- Treatment factors associated with risk of hypertension included abdominal radiation therapy of more than 20 Gy and total-body irradiation (TBI).
- Among childhood cancer survivors, glomerular filtration rate (GFR) of less than 60 mL/min/1.73 m2 was significantly associated with hypertension (OR, 3.4; 95% CI, 1.4–8.5).
- The CCSS demonstrated that the cumulative incidence of serious cardiac events (myocardial infarction, congestive heart failure, pericardial disease, and valvular abnormalities) in childhood cancer survivors continues to increase beyond age 45 years.[7]
- The risk of these events was potentiated (i.e., beyond what would be expected by an additive model) by the presence of concurrent, but potentially modifiable, conditions such as obesity, dyslipidemia, diabetes, and, particularly, hypertension.
- Hypertension was independently associated with all serious cardiac outcomes (rate ratios, 6-fold to 19-fold), even after adjustment for anthracycline use and chest irradiation.
- Of 670 survivors of Hodgkin lymphoma who were treated at St. Jude Children's Research Hospital (SJCRH) and survived 10 or more years, 348 patients were clinically assessed in the SJLIFE cohort study.[23]
- Overall, survivors had a higher cumulative burden (a novel measurement of disease burden that incorporates multiple health conditions and recurrent events into a single metric) than community controls, with the total grade 3 to 5 cumulative burden among survivors at age 30 years being comparable with that of community controls at 50 years.
- At age 50 years, the cumulative incidence of those survivors experiencing at least one grade 3 to grade 5 cardiovascular condition was 45.5% (95% CI, 36.6%–54.3%), compared with 15.7% (95% CI, 7.0%–24.4%) in community controls.
- Myocardial infarction and structural heart defects were the major contributors to the excess grade 3 to grade 5 cumulative burden in survivors, whereas there was no notable difference in survivors and community controls at age 50 years for grades 3 to 5 cumulative burden of dyslipidemia and essential hypertension.
- Another SJLIFE cohort study compared the prevalence of major and minor electrocardiography (ECG) abnormalities among 2,715 participants and 268 community controls.[24]
- Major ECG abnormalities were significantly more prevalent in survivors (10.7%) than in controls (4.9%). The most common abnormalities included isolated ST-T wave abnormalities (7.2%), evidence of myocardial infarction (3.7%), and left ventricular hypertrophy with strain pattern (2.8%).
- Treatment exposures predicting increased risk of major abnormalities were anthracycline doses of 300 mg/m2 or greater (OR, 1.7; 95% CI, 1.1–2.5) and cardiac radiation (OR, 2.1; 95% CI, 1.5–2.9 [0.01–19.99 Gy]; OR, 2.6; 95% CI, 1.6–3.9 [20–29.99 Gy]; OR, 10.5; 95% CI, 6.5–16.9 [≥30 Gy]).
- Major ECG abnormalities were predictive of all-cause mortality (HR, 4.0; 95% CI, 2.1–7.8).
- In the Teenage and Young Adult Cancer Survivor Study, cardiac mortality was investigated in more than 200,000 5-year survivors of adolescent and young adult cancer (aged 15–39 years).[3]
- Age at diagnosis and type of cancer were identified as being important in determining risk of cardiac mortality.
- The SMRs for all cardiac disease combined was greatest for individuals diagnosed at age 15 to 19 years (4.2), decreasing to 1.2 for individuals aged 35 to 39 years (2-sided P for trend < .0001). This age effect was most apparent for survivors of Hodgkin lymphoma, who were also found to be at greatest risk overall.
- Limitations of this study included lack of detailed information on exposures to radiation therapy (doses, fields), exposures to chemotherapy (primarily anthracycline dose), and cardiovascular risk factors (e.g., smoking, obesity, hypertension, diabetes, family history).
- Dutch investigators evaluated the risk of heart failure, temporal changes by treatment periods, and the risk factors for heart failure in 6,165 childhood cancer survivors (median age, 27.3 years; median follow-up, 19.8 years) diagnosed between 1963 and 2002.[25]
- The cumulative incidence of developing a heart failure 40 years after a childhood cancer diagnosis was 4.4% (3.4%–5.5%) among cohort members.
- The 20-year cumulative incidence of grade 3 or higher heart failure was greater among survivors treated in the more recent treatment periods (for survivors diagnosed between 1990 and 2001, 1.5%; between 1980 and 1989, 1.6%; and between 1970 and 1979, 0.5%) than in survivors treated earlier; however, mortality caused by heart failure decreased in the patients who were treated in the more recent periods.
- Multivariable analysis demonstrated that patients who received higher doses of mitoxantrone or cyclophosphamide had an increased risk of heart failure compared with survivors who were exposed to lower doses.
- The Pediatric Normal Tissue Effects in the Clinic (PENTEC) initiative reported on the risk of late cardiac disease for childhood cancer survivors who were treated with chemotherapy or radiation therapy.[26]
- For each 10-Gy increase in corrected mean cardiac radiation dose in 1.8- to 2.0-Gy fractions, the estimated HRs were 2.01 (95% CI, 1.79–2.25) for coronary artery disease, 1.87 (95% CI, 1.70–2.06) for heart failure, 1.87 (95% CI, 1.78–1.96) for valvular disease, and 1.88 (95% CI, 1.75–2.03) for any cardiac disease.
- For each 100-mg/m2 increase in cumulative anthracycline dose, the HR was 1.93 (95% CI, 1.58–2.36) for the development of heart failure, which is equivalent to an increase in mean heart radiation dose of approximately 10.5 Gy.
Treatment Risk Factors
Chemotherapy (in particular, anthracyclines and anthraquinones) and radiation therapy, both independently and in combination, increase the risk of cardiovascular disease in survivors of childhood cancer and are considered to be the most important risk factors contributing to premature cardiovascular disease in this population.[3,9,16,27]
Anthracyclines and related agents
Anthracyclines (e.g., doxorubicin, daunorubicin, idarubicin, and epirubicin) and anthraquinones (e.g., mitoxantrone) are known to directly injure cardiomyocytes through inhibition of topoisomerase 2-beta in cardiomyocytes and formation of reactive oxygen species, resulting in activation of cell-death pathways and inhibition of mitochondrial apoptosis.[28,29] The downstream results of cell death are changes in heart structure, including wall thinning, which leads to ventricular overload and pathological remodeling that, over time, leads to dysfunction and eventual clinical heart failure.[30,31]
Risk factors for anthracycline-related cardiomyopathy include the following:[18,32]
- Cumulative dose, particularly greater than 250 to 300 mg/m2.
- Younger age at time of exposure, particularly children younger than 5 years.
- Increased time from exposure.
- While there is no definitive safe lower dose threshold, doses in excess of 250 to 300 mg/m2 have been associated with a substantially increased risk of cardiomyopathy, with cumulative incidences exceeding 5% after 20 years of follow-up, and in some subgroups, reaching or exceeding 10% cumulative incidence by age 40 years.[17,18,31]
- Concurrent chest or heart radiation therapy also further increases risk of cardiomyopathy,[9,27,33] as does the presence of other cardiometabolic traits such as hypertension.[7,34]
- While development of clinical heart failure can occur within a few years after anthracycline exposure, in most survivors—even those who received very high doses—clinical manifestations may not occur for decades.
Anthracycline dose equivalency
Traditionally, anthracycline dose equivalence has largely been based on acute hematologic toxicity equivalence rather than late cardiac toxicity.
- Analyses that pooled data from more than 28,000 long-term childhood cancer survivors monitored through age 40 years (resulting in 399 cardiomyopathy cases) have challenged previous assumptions that consider daunorubicin equivalent or nearly equivalent to doxorubicin.[35,36]
- These investigations found that daunorubicin may be significantly less cardiotoxic than doxorubicin (equivalence ratio, 0.5; 95% CI, 0.4–0.7).[35]
- Compared with doxorubicin, mitoxantrone may be significantly more cardiotoxic than previously thought (equivalence ratio, 10.5; 95% CI, 6.2–19.1), while epirubicin appeared to be doxorubicin isoequivalent (equivalence ratio, 0.8; 95% CI, 0.3–1.4).[36]
- Data were too sparse to compare idarubicin with doxorubicin.
Anthracycline cardioprotection
Cardioprotective strategies that have been explored include the following:
-
New, less cardiotoxic agents and liposomal formulations. In general, data on whether liposomal formulations of anthracyclines reduce cardiac toxicity in children are limited.[37,38]
-
Prolonged infusion time. Prolonged infusion time has been associated with reduced heart failure in adult patients, but not in children.[39,40]
-
Concurrent administration of cardioprotectants. A variety of agents have been tested as cardioprotectants (amifostine, acetylcysteine, calcium channel blockers, carvedilol, coenzyme Q10, and L-carnitine), but none were beneficial and are not considered standard of care.[41,42]
-
Dexrazoxane. There are more data for dexrazoxane as a cardioprotectant, but mainly in adult patients with cancer. In particular, the U.S. Food and Drug Administration approved dexrazoxane for women with metastatic breast cancer who have received 300 mg/m2 of anthracyclines and who may benefit from further anthracycline-based therapy.[41]
- Pediatric data show that dexrazoxane may ameliorate some markers of early cardiac toxicity for up to 5 years after therapy.[43,44,45,46]
- Dexrazoxane may be associated with an increased risk of acute toxicities in some regimens.[47]
- A long-term study assessed outcomes of newly diagnosed children with cancer who were treated with dexrazoxane-containing regimens in randomized clinical trials (cumulative prescribed doxorubicin dose, 100–360 mg/m2; median follow-up, 18.6 years). Dexrazoxane was not associated with cancer relapse (HR, 0.84; 95% CI, 0.63–1.13), second cancers (HR, 1.19; 95% CI, 0.62–2.30), all-cause mortality (HR, 1.07; 95% CI, 0.78–1.47), or cardiovascular mortality (HR, 1.45; 95% CI, 0.41–5.16).[48]
- Another study evaluated long-term cardiac outcomes of 195 children with acute lymphoblastic leukemia or Hodgkin lymphoma who received doxorubicin with or without dexrazoxane in four randomized trials and patients with osteosarcoma who all received doxorubicin with dexrazoxane in a nonrandomized trial.[49]
- At 18.1 years since cancer diagnosis (51% dexrazoxane exposed; cumulative doxorubicin dose, 297 mg/m2), dexrazoxane administration was associated with superior left ventricular fractional shortening (absolute difference, 1.4%; 95% CI, 0.3–2.5) and ejection fraction (absolute difference, 1.6%; 95% CI, 0.0–3.2) and lower myocardial stress per B-type natriuretic peptide (-6.7 pg/mL; 95% CI, -10.6 to -2.8).
- Dexrazoxane use was associated with a reduced risk of lower left ventricular function (30% fractional shortening or 50% ejection fraction; OR, 0.24; 95% CI, 0.07–0.81), which was primarily observed in those treated with cumulative doxorubicin doses of 250 mg/m2 or higher.
Radiation therapy
While anthracyclines directly damage cardiomyocytes, radiation therapy primarily affects the fine vasculature of affected organs.[6]
Cardiovascular disease
Late effects of radiation therapy to the heart specifically include the following:
- Delayed pericarditis, which can present abruptly or as a chronic pericardial effusion.
- Pancarditis that includes pericardial and myocardial fibrosis, with or without endocardial fibroelastosis.
- Cardiomyopathy (in the absence of significant pericardial disease), which can occur even without anthracycline exposure.
- Ischemic heart disease.
- Functional valve injury, often aortic.
- Conduction defects.
These cardiac late effects are related to the following:
- Individual radiation fraction size.
- Volume of the heart that is exposed to radiation.[18,50]
- Total radiation dose.
- Various studies have demonstrated a substantially increased risk of these outcomes with higher radiation doses, particularly doses to the heart exceeding 35 Gy.[9,11,17,18,27]
- At higher radiation doses, rates of heart failure, pericardial disease, and valvular disease have been reported to exceed 10% after 20 to 30 years. Although some studies suggest that doses less than 5 Gy may be associated with an increased risk of cardiovascular disease, the relative risk is small (i.e., 2.5) and the 95% CI is large (i.e., 0.2–41.5); moreover, the dosimetric analyses are generally estimations of incidental cardiac exposure.[9,17,27]
- Low to moderate doses of radiation therapy (5.0–19.9 Gy) to large cardiac volumes (>50% of the heart) are associated with an increased rate of cardiac disease (i.e., 1.6-fold) compared with survivors who did not have any cardiac radiation therapy exposure.[18]
- High doses of radiation (>20 Gy) to small cardiac volumes (0.1%–29.9%) are associated with an elevated rate of cardiac disease (relative rate, 2.4).[18,51]
- Additional confirmatory data are needed for an accurate assessment of risk at very low cardiac doses.
- Similar to anthracyclines, manifestation of these late effects may take years, if not decades, to present.
Patients who were exposed to both radiation therapy affecting the cardiovascular system and cardiotoxic chemotherapy agents are at even greater risk of late cardiovascular outcomes.[9,18] This risk may be decreasing based on Children's Oncology Group (COG) Hodgkin lymphoma clinical trials spanning from 2002 to 2022.[52]
- In a cohort study of 2,563 patients with Hodgkin lymphoma treated in four consecutive COG clinical trials between 2002 and 2022 (AHOD0031, AHOD0831, AHOD1331, S1826), the cumulative incidence of grades 3 to 5 cardiac disease was estimated to decrease, from 10% in the first trial to 6% in the last trial.
- All patients received doxorubicin, 1,362 received mediastinal radiation therapy, and 307 received the cardioprotectant dexrazoxane (80% of children in the S1826 study). Radiation therapy was substantially reduced and refined, whereas the anthracycline doses were increased.
- Based on modeling used as part of this study, survivors treated in the recent high-risk Hodgkin lymphoma trial (S1826), the 30-year risk of serious cardiac morbidity will be increased less than 2% above the expected rate in an untreated population (5%). Reductions in the proportion of children receiving mediastinal radiation therapy and increases in dexrazoxane use were estimated to offset the increased doxorubicin dose, producing a net reduction in late cardiac disease.
- These results suggest that cardiac toxic effects are estimated to decrease from older to newer Hodgkin lymphoma trials, even in females aged 12 years and younger, who are most susceptible to anthracycline-induced heart failure.
Cerebrovascular disease
Cerebrovascular disease after radiation therapy exposure is another potential late effect observed in survivors.
- Radiation-induced vascular damage is a complex process that involves both arterial and capillary damage, with veins being less sensitive.
- The spectrum of abnormalities includes lacunar lesions, vascular malformations, telangiectasias, intracranial hemorrhage, moyamoya, microbleeds, and cavernomas, each with potential symptomatic consequences.[53,54]
- While brain tumor survivors have traditionally had the greatest risk, other survivors exposed to cranial irradiation (≥18 Gy) and neck irradiation (≥40 Gy), such as leukemia and lymphoma survivors, have also been reported to be at increased risk.[55,56,57,58]
- In a PENTEC analysis, risk of cerebrovascular toxicity and future risk of stroke were analyzed and modeled (COX proportional hazards and baseline cumulative incidences of stroke in nonradiation therapy exposed, age-matched cancer patients and the general population).[59]
- Of 3,898 pediatric patients analyzed in five reports, 101 experienced at least one cerebrovascular toxicity (e.g., transient ischemic attack [TIA], stroke, moyamoya, or arteriopathy).
- The risk of any cerebrovascular toxicity was 0.2% at 30 Gy, 1.3% at 45 Gy, and 4.4% at 54 Gy (D50, 75.6 Gy; 68.4–89.4 Gy).
- At an attained age of 35 years, the predicted stroke incidence at d doses was 0.9% to 1.3% at 30 Gy, 1.8% to 2.7% at 45 Gy, and 2.8% to 4.1% at 54 Gy (population baseline risk, 0.2%–0.3%).
- At an attained age of 45 years, the predicted stroke incidence was 2.1% to 4.2% at 30 Gy, 4.5% to 8.6% at 45 Gy, and 6.7% to 13.0% at 54 Gy (population baseline risk, 0.5%–1.0%).
- Thus, the risk of future cerebrovascular toxicity continues to rise as follow-up duration increases. Additionally, because the stroke hazard was based on prescribed tumor bed dose rather than circle-of-Willis dose, the reported stroke risk was likely underestimated.
- In lymphoma survivors who received only chest and/or neck radiation therapy, cerebrovascular disease is thought to be caused by large-vessel atherosclerosis and cardiac embolism.[56]
- The risk increases with cumulative dose received. One study (N = 325) reported that the stroke hazard increased by 5% (HR, 1.05; 95% CI, 1.01–1.09; P = .02), with each 1 Gy increase in the radiation dose, leading to a cumulative incidence of 2% for the first stroke after 5 years and 4% after 10 years.[60]
- Survivors who experienced a stroke were at much greater risk of recurrent strokes.[61]
Evidence (selected studies describing prevalence of and risk factors for cerebrovascular accident [CVA]/vascular disease):
- The population-based British Childhood Cancer Survivor Study (n = 13,457) used Hospital Episode Statistics data for England to assess the risk of cerebrovascular-related hospitalizations (e.g., nontraumatic intracranial hemorrhage, cerebral infarction, or cerebral artery occlusion), particularly in patients older than 50 years.[58]
- They found that 2.3% of the survivors had been hospitalized at least once for cerebrovascular disease, with a fourfold risk compared with the expected rate.
- Survivors of central nervous system (CNS) tumors or leukemia who received cranial irradiation were at greatest risk of cerebrovascular disease (CNS tumor standardized hospitalization ratio [SHR], 15.6; 95% CI, 14.0–17.4 and leukemia SHR, 5.4; 95% CI, 4.5–6.4).
- Beyond age 60 years, on average, 3.1% of CNS tumor survivors treated with cranial irradiation were hospitalized annually for cerebrovascular disease. By age 65 years, as many as 26% of these patients will have been hospitalized for a cerebrovascular event.
- CCSS investigators observed that 295 of 13,060 participants (35% treated with cranial radiation therapy) reported having a stroke (6.3%; 95% CI, 5.1%–7.5%) by age 50 years (median follow-up, 19 years). For survivors considered to be high risk, the cumulative incidence of cerebrovascular disease up to age 50 was 19.9%.[62]
- A retrospective study of 3,172 5-year survivors of childhood cancer monitored for a mean time of 26 years was formed from the Euro2K cohort, which included eight centers in France and the United Kingdom. Radiation doses to the circle of Willis were estimated for each of the 2,202 children who received radiation therapy.[63]
- Patients who received radiation therapy had an 8.5-fold increased risk (95% CI, 6.3–11.0) of stroke in contrast to a nonelevated risk for patients who did not receive radiation therapy.
- The relative risk was 15.7 (95% CI, 4.9–50.2) for doses of 40 Gy or higher.
- At age 45 years, the cumulative incidence was 11.3% (95% CI, 7.1%–17.7%) in patients who received 10 Gy or higher to the circle of Willis, compared with 1% in the general population.
- Investigators from the Teenage and Young Adult Cancer Survivor Study (N = 178,962) evaluated the risk of hospitalization for a cerebrovascular event among 5-year survivors of cancer diagnosed between age 15 and 39 years.[64]
- The investigators found that survivors of adolescent and young adult cancers had a 40% increased risk of hospitalization for cerebrovascular event compared with the general population.
- Survivors of CNS tumors (SHR, 4.6), head and neck tumors (SHR, 2.6), and leukemia (SHR, 2.5) had the highest risk of hospitalization for a cerebrovascular complication.
- Males had significantly higher absolute excess risks than did females, especially among head and neck tumor survivors. By age 60 years, 9% of CNS tumor survivors, 6% of head and neck tumor survivors, and 5% of leukemia survivors had been hospitalized for a cerebrovascular event.
- The risk of hospitalization for a cerebral infarction was particularly increased among survivors of a CNS tumor older than 60 years, whereas this risk was increased across all ages in survivors of head and neck tumors.
- CCSS investigators evaluated the rates and predictors of recurrent stroke among participants who reported a first stroke.[61]
- Among responding participants (329 of 443), 271 confirmed a first stroke (at median age, 19 years) and 70 reported a second stroke (at median age, 32 years).
- Independent predictors of recurrent stroke included treatment with a cranial radiation therapy dose of 50 Gy or higher (vs. no cranial radiation therapy), history of hypertension, and age 40 years or older at first stroke (vs. age 0–17 years).
- The 10-year cumulative incidence of late recurrent stroke was 21% overall and 33% for those treated with 50 Gy or higher of cranial radiation therapy.
- A follow-up study of 224 CCSS participants who experienced stroke demonstrated an increased risk of all-cause and health-related mortality and a negative impact on social attainment, neurocognitive function, emotional distress, and other health-related quality-of-life measures.[65]
- A multicenter retrospective Dutch study evaluated 5-year survivors of Hodgkin lymphoma diagnosed before age 51 years (25% pediatric-aged patients) who were followed for a median of 18 years. Among 2,201 survivors, 96 developed cerebrovascular disease (CVA and TIA).[56]
- Most ischemic events were from large-artery atherosclerosis (36%) or cardiac embolism (24%).
- The cumulative incidence of ischemic CVA or TIA 30 years after lymphoma treatment was 7%.
- The overall standardized incidence ratio (SIR) was 2.2 for CVA and 3.1 for TIA. However, SIR estimates appeared to be greater among childhood cancer survivors, with SIRs of 3.8 for CVA and 7.6 for TIA.
- Irradiation to the neck and mediastinum was an independent risk factor for ischemic cerebrovascular disease (HR, 2.5; 95% CI, 1.1–5.6) versus no radiation therapy. Treatment with chemotherapy was not associated with increased risk.
- Hypertension, diabetes mellitus, and hypercholesterolemia were associated with the occurrence of ischemic cerebrovascular disease.
Venous thromboembolism
Children with cancer have an excess risk of venous thromboembolism within the first 5 years after diagnosis. However, the long-term risk of venous thromboembolism among childhood cancer survivors has not been well studied.[66]
CCSS investigators evaluated self-reported late-onset (5 or more years after cancer diagnosis) venous thromboembolism among cohort members (median follow-up, 21.3 years).[67]
- The 35-year cumulative incidence of venous thromboembolism among survivors was 4.9%. This risk was twofold higher when compared with a sibling cohort (rate ratio, 2.2; 95% CI, 1.7–2.8).
- Risk factors for venous thromboembolism among survivors included female sex, treatment with cisplatin or asparaginase, being affected by obesity or underweight, and recurrent primary or subsequent cancer.
- The risk of late venous thromboembolism was higher among survivors of lower-extremity osteosarcoma treated with limb-sparing surgery compared with patients treated with amputation, possibly resulting from alterations in peripheral vascular anatomy and homeostasis.
- Venous thromboembolism was associated with an almost-twofold increased risk of late mortality (RR, 1.9; 95% CI, 1.6–2.3).
Conventional cardiovascular conditions
- Various cancer treatment exposures may also directly or indirectly influence the development of hypertension, diabetes mellitus, and dyslipidemia.
- These conditions remain important among cancer survivors, as they do in the general population, in that they are independent risk factors in the development of cardiomyopathy, ischemic heart disease, and cerebrovascular disease.[7,68,69,70,71]
- Childhood cancer survivors should be closely monitored for the development of these cardiovascular conditions because they represent potentially modifiable targets for intervention.
- Related conditions such as obesity and various endocrinopathies (e.g., hypothyroidism, hypogonadism, growth hormone deficiency) that may be more common among subsets of childhood cancer survivors also need to be monitored. If these conditions are untreated/uncontrolled, they may be associated with a metabolic profile that increases cardiovascular risk.[8] For more information, see the Risk prediction for cardiovascular diseases section.
Other Risk Factors
-
Sex. Some, but not all, studies suggest that female sex may be associated with a greater risk of anthracycline-related cardiomyopathy.[6]
-
Genetics. There is emerging evidence that genetic factors, such as single nucleotide polymorphisms in genes regulating drug metabolism and distribution, could explain the heterogeneity in susceptibility to anthracycline-mediated cardiac injury.[72,73,74,75,76,77,78,79,80] However, these genetic findings still require additional validation before integration into any clinical screening algorithm.[80]
Peripartum Cardiac Dysfunction
Long-term survivors of childhood, adolescent, and young adult malignancies with past exposure to potentially cardiotoxic treatments are at risk of peripartum cardiac dysfunction.
In the general population, peripartum cardiomyopathy (PPCM) is a rare condition characterized by heart failure during pregnancy (usually the last trimester or <5 months postpartum). The estimated incidence in the general population is 1 case per 3,000 live births.[81]
There are limited data available about the prevalence in survivors of pediatric, adolescent, and young adult malignancies who have received cardiotoxic therapies.
- In a retrospective series from SJCRH, 3 cases of peripartum cardiac dysfunction occurred in 1,554 completed pregnancies, which was an incidence of 0.2%; 27% of the 847 long-term survivors had not been exposed to cardiotoxic therapies.[82]
- In a series of 64 women who had all received cardiotoxic therapy (44% received chest radiation therapy plus anthracyclines, 14% received chest radiation therapy, 42% received anthracycline alone), 5 women (7.8%) had peripartum cardiac events (3 symptomatic, 2 subclinical). Of the 110 live births, 2 involved PPCM, representing a 55-fold increased risk over the general population. Risk factors were younger age at cancer diagnosis and higher anthracycline dose. Postpartum cardiac function failed to return to baseline in four women (80%).[83]
- A single-center study estimated the risk of developing congestive heart failure (CHF) during pregnancy as 1 out of 3 female cancer survivors with a history of cardiotoxicity.[84] Seventy-eight consecutive female cancer survivors previously exposed to potentially cardiotoxic treatments (chemotherapy and/or radiation therapy to the thorax as children, adolescents or young adults) who had 94 pregnancies were retrospectively identified through high-risk clinics between 2005 and 2015.
- A total of 55 women received anthracyclines (range, 90–500 mg/m2), while 23 received nonanthracycline chemotherapy and/or radiation therapy only. Of the 13 women with prior cardiotoxicity, 8 had reduced left ventricular ejection fraction at the first antenatal visit. CHF occurred in five pregnancies (4 women; 5.3%).
- The incidence of CHF was 31% for women with a history of cardiotoxicity and 0% for women without a history of cardiotoxicity. When comparing clinical characteristics between women with and without CHF, there was no difference in age of cancer diagnosis, cancer type, or exposure to anthracyclines.
- Risk factors for developing CHF were history of cardiotoxicity before pregnancy, left ventricular systolic dysfunction at first antenatal visit, or receiving cardiac medications.
Based on available evidence about peripartum cardiomyopathy, the International Guideline Harmonization Group assessed that cardiomyopathy surveillance is reasonable before pregnancy or in the first trimester for female survivors of childhood, adolescent, and young adult cancer who are at moderate and high risk because they were treated with anthracyclines or chest radiation therapy.[32]
Mortality Risk After Major Cardiovascular Events
Survivors of childhood cancer represent a population at high risk of mortality after major cardiovascular events. Investigators estimated the cumulative incidence of all-cause and cardiovascular cause–specific mortality among survivors from the CCSS who had experienced a major cardiovascular event and compared them to siblings. They also compared the outcomes from the CCSS cancer survivors with a population-based cohort of racially diverse adults from the Coronary Artery Risk Development in Young Adults (CARDIA) study.[85]
- Among the 25,658 childhood cancer survivors (median age at diagnosis, 7 years; median age at follow-up or death, 38 years) and 5,051 siblings, 1,780 survivors and 91 siblings experienced a cardiovascular event.
- The 10-year all-cause mortality rate for survivors was 30% after heart failure, 36% after cardiovascular artery disease, and 29% after stroke. The 10-year all-cause mortality rate for siblings was 14% after heart failure, 14% after coronary artery disease, and 4% after stroke (P < .001 for all survivors).
- All-cause mortality risks among childhood cancer survivors were increased after heart failure (HR, 7.32), coronary artery disease (HR, 5.54), and stroke (HR, 3.57).
- Among 5,114 CARDIA participants, 345 had a major event. CARDIA participants were, on average, decades older at events (median age, 57 years vs. 31 years). However, mortality risks were similar, except that all-cause mortality after coronary artery disease was significantly increased among childhood cancer survivors (HR, 1.85).
Heart Transplant After Childhood Cancer
Data about the prevalence and outcomes of survivors with heart failure requiring heart transplant are limited.
- In a study of solid organ transplants in 13,318 survivors in the CCSS, 62 survivors had end-stage heart failure that warranted heart transplants, 37 of whom received a heart transplant.[86]
- At 35 years after cancer diagnosis, the cumulative incidence of heart transplant was 0.30%, and the cumulative incidence of being placed on the waiting list or receiving a heart was 0.49%.[86]
- The 5-year survival rate from heart transplant was 80.6%, which is similar to the outcome in the general population of the same age range.[86]
Knowledge Deficits
While much knowledge has been gained over the past 20 years in better understanding the long-term burden and risk factors for cardiovascular disease among childhood cancer survivors, many areas of inquiry remain, and include the following:
- Radiation may have both direct and indirect effects on vascular endothelium, contributing to vascular damage beyond the primary radiation field.[87]
- The long-term effects of lower radiation doses, particularly in the setting of advanced technology that allows tumor targeting from multiple directions and reduces exposure to surrounding normal tissues, remain to be determined.[88]
- The long-term effects of many newer anticancer agents that are based on molecular targets remain unclear, although some of them are known to have shorter-term cardiac toxicity.[15]
- The efficacy of cardioprotective strategies, including the use of alternative anthracycline formulations that appear promising in adults, requires further study in children.[42]
Screening, Surveillance, and Counseling
The International Guideline Harmonization Group has worked collaboratively to harmonize evidence-based cardiac surveillance recommendations and have identified knowledge deficits to help guide future studies.[32,71] Risk groups defined by cumulative exposures of anthracycline and chest-directed radiation therapy as well as cardiomyopathy surveillance recommendations are summarized in Table 2.
Consensus regarding evidence about screening, surveillance, and counseling
- There is no clear evidence (at least through age 50 years or 30–40 years posttreatment) that a plateau in risk occurs after a certain time among survivors exposed to cancer treatments associated with cardiovascular late effects.[89,90] Thus, life-long surveillance is recommended by one group, even if the cost-effectiveness of certain screening strategies remains unclear.[32,91,92,93]
Table 2. Risk Groups and Cardiomyopathy Surveillance Recommendations for Survivors of Childhood, Adolescent, and Young Adult CanceraRisk Group | Anthracycline (mg/m2) | Chest-Directed Radiation Therapy (Gy) | Anthracycline (mg/m2) + Chest-Directed Radiation Therapy (Gy) | Is Screening Recommended? | At What Interval? |
---|
NA = not applicable. |
a Adapted from Ehrhardt et al.[32] |
High risk | ≥250 | ≥30 | ≥100 and ≥15 | Yes | 2 years |
Moderate risk | 100 to <250 | 15 to <30 | NA | Maybe | 5 years |
Low risk | >0 to <100 | >0 to <15 | NA | No | No screening |
- A growing body of literature is beginning to establish the yield from these screening studies, which will help inform future guidelines.[8,94,95,96] In these studies, for example, among adult-aged survivors of childhood cancer, evidence for cardiomyopathy on the basis of echocardiographic changes was found in approximately 6% of at-risk survivors. Overall, in a cohort of more than 1,000 survivors (median age, 32 years), nearly 60% of screened at-risk survivors had some clinically ascertained cardiac abnormality identified.[8]
- Given the growing evidence that conventional cardiovascular conditions such as hypertension, dyslipidemia, and diabetes substantially increase the risk of more serious cardiovascular disease among survivors, clinicians should carefully consider baseline and follow-up screening and treatment of these comorbid conditions that impact cardiovascular health (see Table 3).[7,56,68,97]
- There is also emerging evidence that adoption of healthier lifestyle factors may decrease future cardiovascular morbidity in at-risk survivors.[98,99] Thus, similar to the general population, survivors should be counseled about maintaining a healthy weight, participating in regular physical activity, adhering to a heart-healthy diet, and abstaining from smoking.
- The COG has organized handouts on cardiovascular disease and related topics, including lifestyle choices, written for a lay audience to facilitate counseling and education of survivors. For more information, see the COG Survivorship Guidelines.
Predicting Cardiovascular Disease Risk
- Attempts to develop more individualized risk prediction for cardiovascular disease may help refine surveillance and counseling in the future.
- Several groups have collaborated to develop and validate individualized risk calculators for heart failure, ischemic heart disease, and stroke through age 50 years.[33,62,97]
- Updated models based only on CCSS data have incorporated hypertension, dyslipidemia, and diabetes status across time to further refine prediction.[97]
- An online risk calculator incorporating these models is available on the CCSS website.
Risk prediction and interventions for cardiovascular diseases
- Using data from four large, well-annotated childhood cancer survivor cohorts (CCSS, National Wilms Tumor Study Group, the Netherlands, and SJCRH), a heart failure risk calculator based on readily available demographic and treatment characteristics has been created and validated. This calculator may provide more individualized clinical heart failure risk estimation for 5-year survivors of childhood cancer who have recently completed therapy, through age 40 years. Because of the young age of participants at the time of baseline prediction (5-year survival), this estimator is limited in that information on conventional cardiovascular conditions such as hypertension, dyslipidemia, or diabetes could not be incorporated.[33]
- In another collaborative study, data from the CCSS, Netherlands, and SJCRH were used to develop risk-prediction models for ischemic heart disease and stroke among 5-year survivors of childhood cancer through age 50 years. Risk scores derived from a standard prediction model that included sex, chemotherapy exposure, and radiation therapy exposure identified statistically distinct low-risk, moderate-risk, and high-risk groups. The cumulative incidences at age 50 years among CCSS low-risk groups were less than 5%, compared with approximately 20% for high-risk groups and only 1% for siblings.[62]
- Traditional cardiovascular risk factors remain important for predicting risk of cardiovascular disease among adult-aged survivors of childhood cancer. This finding was demonstrated by a CCSS investigation that constructed prediction models accounting for cardiotoxic cancer treatment exposures, combined with information on traditional cardiovascular risk factors such as hypertension, dyslipidemia, and diabetes. Risk scores based on demographic, cancer treatment, hypertension, dyslipidemia, and diabetes information showed good performance (area under the receiver operating characteristic curve and concordance statistics ≥0.70) for predicting cardiovascular events in the models applied to the discovery and replication cohorts. The most influential exposures were anthracycline chemotherapy, radiation therapy, diabetes, and hypertension.[97]
- Physical activity has been shown to be a safe intervention against the high burden of cardiovascular late effects in childhood cancer survivors. A randomized controlled trial investigated the effect of a partially supervised, personalized, 1-year physical activity intervention on cardiovascular health in long-term survivors of childhood cancer.[100] There were 151 childhood cancer survivors randomly assigned (1:1) to either perform more than 2.5 hours of additional intense physical activity per week (intervention group) or continue exercise as usual (control group). A significant and robust reduction of the cardiovascular disease risk score was noted at 6 months and 12 months in the intervention group, compared with the control group. The difference in the reduction of the cardiovascular disease risk z-score of -0.18 (P = .003) at 12 months favored the intervention group.
Table 3. Cardiovascular Late Effectsa,b
Predisposing Therapy |
Potential Cardiovascular Effects |
Health Screening |
a The Children's Oncology Group (COG) guidelines also cover other conditions that may influence cardiovascular risk, such as obesity and diabetes mellitus/impaired glucose metabolism. |
b Adapted from theChildren's Oncology Group Long-Term Follow-Up Guidelines for Survivors of Childhood, Adolescent, and Young Adult Cancers. |
Any anthracycline and/or any radiation exposing the heart |
Cardiac toxicity (arrhythmia, cardiomyopathy/heart failure, pericardial disease, valve disease, ischemic heart disease) |
Yearly medical history and physical examination |
Electrocardiography at entry into long-term follow-up |
Echocardiography at entry into long-term follow-up, periodically repeat based on previous exposures and other risk factors |
Radiation exposing the neck and base of skull (especially ≥40 Gy) |
Carotid and/or subclavian artery disease |
Yearly medical history and physical examination; consider Doppler ultrasonography 10 years after exposure |
Radiation exposing the brain/cranium (especially ≥18 Gy) |
Cerebrovascular disease (cavernomas, moyamoya, occlusive cerebral vasculopathy, stroke) |
Yearly medical history and physical examination |
Radiation exposing the abdomen |
Diabetes |
Diabetes screening every 2 years |
Total-body irradiation (usually <14 Gy) |
Dyslipidemia; diabetes |
Fasting lipid profile and diabetes screening every 2 years |
Heavy metals (carboplatin, cisplatin), and ifosfamide exposure; radiation exposing the kidneys; HSCT; nephrectomy |
Hypertension (from renal toxicity) |
Yearly blood pressure test; renal function laboratory studies at entry into long-term follow-up and repeat as clinically indicated |
HSCT = hematopoietic stem cell transplant. |
References:
-
Armstrong GT, Chen Y, Yasui Y, et al.: Reduction in Late Mortality among 5-Year Survivors of Childhood Cancer. N Engl J Med 374 (9): 833-42, 2016.
-
Fidler MM, Reulen RC, Winter DL, et al.: Long term cause specific mortality among 34 489 five year survivors of childhood cancer in Great Britain: population based cohort study. BMJ 354: i4351, 2016.
-
Henson KE, Reulen RC, Winter DL, et al.: Cardiac Mortality Among 200 000 Five-Year Survivors of Cancer Diagnosed at 15 to 39 Years of Age: The Teenage and Young Adult Cancer Survivor Study. Circulation 134 (20): 1519-1531, 2016.
-
Suh E, Stratton KL, Leisenring WM, et al.: Late mortality and chronic health conditions in long-term survivors of early-adolescent and young adult cancers: a retrospective cohort analysis from the Childhood Cancer Survivor Study. Lancet Oncol 21 (3): 421-435, 2020.
-
Fidler MM, Reulen RC, Henson K, et al.: Population-Based Long-Term Cardiac-Specific Mortality Among 34 489 Five-Year Survivors of Childhood Cancer in Great Britain. Circulation 135 (10): 951-963, 2017.
-
Bansal N, Blanco JG, Sharma UC, et al.: Cardiovascular diseases in survivors of childhood cancer. Cancer Metastasis Rev 39 (1): 55-68, 2020.
-
Armstrong GT, Oeffinger KC, Chen Y, et al.: Modifiable risk factors and major cardiac events among adult survivors of childhood cancer. J Clin Oncol 31 (29): 3673-80, 2013.
-
Hudson MM, Ness KK, Gurney JG, et al.: Clinical ascertainment of health outcomes among adults treated for childhood cancer. JAMA 309 (22): 2371-81, 2013.
-
Haddy N, Diallo S, El-Fayech C, et al.: Cardiac Diseases Following Childhood Cancer Treatment: Cohort Study. Circulation 133 (1): 31-8, 2016.
-
Cotton CA, Peterson S, Norkool PA, et al.: Early and late mortality after diagnosis of wilms tumor. J Clin Oncol 27 (8): 1304-9, 2009.
-
Schellong G, Riepenhausen M, Bruch C, et al.: Late valvular and other cardiac diseases after different doses of mediastinal radiotherapy for Hodgkin disease in children and adolescents: report from the longitudinal GPOH follow-up project of the German-Austrian DAL-HD studies. Pediatr Blood Cancer 55 (6): 1145-52, 2010.
-
Green DM, Kun LE, Matthay KK, et al.: Relevance of historical therapeutic approaches to the contemporary treatment of pediatric solid tumors. Pediatr Blood Cancer 60 (7): 1083-94, 2013.
-
Hudson MM, Neglia JP, Woods WG, et al.: Lessons from the past: opportunities to improve childhood cancer survivor care through outcomes investigations of historical therapeutic approaches for pediatric hematological malignancies. Pediatr Blood Cancer 58 (3): 334-43, 2012.
-
de Baat EC, van Dalen EC, Mulder RL, et al.: Primary cardioprotection with dexrazoxane in patients with childhood cancer who are expected to receive anthracyclines: recommendations from the International Late Effects of Childhood Cancer Guideline Harmonization Group. Lancet Child Adolesc Health 6 (12): 885-894, 2022.
-
Chow EJ, Antal Z, Constine LS, et al.: New Agents, Emerging Late Effects, and the Development of Precision Survivorship. J Clin Oncol 36 (21): 2231-2240, 2018.
-
de Baat EC, Feijen EAM, Reulen RC, et al.: Risk Factors for Heart Failure Among Pan-European Childhood Cancer Survivors: A PanCareSurFup and ProCardio Cohort and Nested Case-Control Study. J Clin Oncol 41 (1): 96-106, 2023.
-
Mulrooney DA, Hyun G, Ness KK, et al.: Major cardiac events for adult survivors of childhood cancer diagnosed between 1970 and 1999: report from the Childhood Cancer Survivor Study cohort. BMJ 368: l6794, 2020.
-
Bates JE, Howell RM, Liu Q, et al.: Therapy-Related Cardiac Risk in Childhood Cancer Survivors: An Analysis of the Childhood Cancer Survivor Study. J Clin Oncol 37 (13): 1090-1101, 2019.
-
Bates JE, Shrestha S, Liu Q, et al.: Cardiac Substructure Radiation Dose and Risk of Late Cardiac Disease in Survivors of Childhood Cancer: A Report From the Childhood Cancer Survivor Study. J Clin Oncol 41 (22): 3826-3838, 2023.
-
Chow EJ, Chen Y, Armstrong GT, et al.: Underdiagnosis and Undertreatment of Modifiable Cardiovascular Risk Factors Among Survivors of Childhood Cancer. J Am Heart Assoc 11 (12): e024735, 2022.
-
Goldberg JF, Hyun G, Ness KK, et al.: Dyslipidemia and cardiovascular disease among childhood cancer survivors: a St. Jude Lifetime Cohort report. J Natl Cancer Inst 116 (3): 408-420, 2024.
-
Kooijmans ECM, van der Pal HJH, Pluijm SMF, et al.: Hypertension in long-term childhood cancer survivors after treatment with potentially nephrotoxic therapy; DCCSS-LATER 2: Renal study. Eur J Cancer 172: 287-299, 2022.
-
Bhakta N, Liu Q, Yeo F, et al.: Cumulative burden of cardiovascular morbidity in paediatric, adolescent, and young adult survivors of Hodgkin's lymphoma: an analysis from the St Jude Lifetime Cohort Study. Lancet Oncol 17 (9): 1325-34, 2016.
-
Mulrooney DA, Soliman EZ, Ehrhardt MJ, et al.: Electrocardiographic abnormalities and mortality in aging survivors of childhood cancer: A report from the St Jude Lifetime Cohort Study. Am Heart J 189: 19-27, 2017.
-
Feijen EAML, Font-Gonzalez A, Van der Pal HJH, et al.: Risk and Temporal Changes of Heart Failure Among 5-Year Childhood Cancer Survivors: a DCOG-LATER Study. J Am Heart Assoc 8 (1): e009122, 2019.
-
Bates JE, Rancati T, Keshavarz H, et al.: Cardiac Disease in Childhood Cancer Survivors Treated With Radiation Therapy: A PENTEC Comprehensive Review. Int J Radiat Oncol Biol Phys 119 (2): 522-532, 2024.
-
van der Pal HJ, van Dalen EC, van Delden E, et al.: High risk of symptomatic cardiac events in childhood cancer survivors. J Clin Oncol 30 (13): 1429-37, 2012.
-
Zhang S, Liu X, Bawa-Khalfe T, et al.: Identification of the molecular basis of doxorubicin-induced cardiotoxicity. Nat Med 18 (11): 1639-42, 2012.
-
Lyu YL, Kerrigan JE, Lin CP, et al.: Topoisomerase IIbeta mediated DNA double-strand breaks: implications in doxorubicin cardiotoxicity and prevention by dexrazoxane. Cancer Res 67 (18): 8839-46, 2007.
-
Hudson MM, Rai SN, Nunez C, et al.: Noninvasive evaluation of late anthracycline cardiac toxicity in childhood cancer survivors. J Clin Oncol 25 (24): 3635-43, 2007.
-
van der Pal HJ, van Dalen EC, Hauptmann M, et al.: Cardiac function in 5-year survivors of childhood cancer: a long-term follow-up study. Arch Intern Med 170 (14): 1247-55, 2010.
-
Ehrhardt MJ, Leerink JM, Mulder RL, et al.: Systematic review and updated recommendations for cardiomyopathy surveillance for survivors of childhood, adolescent, and young adult cancer from the International Late Effects of Childhood Cancer Guideline Harmonization Group. Lancet Oncol 24 (3): e108-e120, 2023.
-
Chow EJ, Chen Y, Kremer LC, et al.: Individual prediction of heart failure among childhood cancer survivors. J Clin Oncol 33 (5): 394-402, 2015.
-
Armenian SH, Sun CL, Vase T, et al.: Cardiovascular risk factors in hematopoietic cell transplantation survivors: role in development of subsequent cardiovascular disease. Blood 120 (23): 4505-12, 2012.
-
Feijen EA, Leisenring WM, Stratton KL, et al.: Equivalence Ratio for Daunorubicin to Doxorubicin in Relation to Late Heart Failure in Survivors of Childhood Cancer. J Clin Oncol 33 (32): 3774-80, 2015.
-
Feijen EAM, Leisenring WM, Stratton KL, et al.: Derivation of Anthracycline and Anthraquinone Equivalence Ratios to Doxorubicin for Late-Onset Cardiotoxicity. JAMA Oncol 5 (6): 864-871, 2019.
-
van Dalen EC, Michiels EM, Caron HN, et al.: Different anthracycline derivates for reducing cardiotoxicity in cancer patients. Cochrane Database Syst Rev (5): CD005006, 2010.
-
Krauss AC, Gao X, Li L, et al.: FDA Approval Summary: (Daunorubicin and Cytarabine) Liposome for Injection for the Treatment of Adults with High-Risk Acute Myeloid Leukemia. Clin Cancer Res 25 (9): 2685-2690, 2019.
-
van Dalen EC, van der Pal HJ, Caron HN, et al.: Different dosage schedules for reducing cardiotoxicity in cancer patients receiving anthracycline chemotherapy. Cochrane Database Syst Rev (4): CD005008, 2009.
-
Lipshultz SE, Giantris AL, Lipsitz SR, et al.: Doxorubicin administration by continuous infusion is not cardioprotective: the Dana-Farber 91-01 Acute Lymphoblastic Leukemia protocol. J Clin Oncol 20 (6): 1677-82, 2002.
-
Hensley ML, Hagerty KL, Kewalramani T, et al.: American Society of Clinical Oncology 2008 clinical practice guideline update: use of chemotherapy and radiation therapy protectants. J Clin Oncol 27 (1): 127-45, 2009.
-
van Dalen EC, Caron HN, Dickinson HO, et al.: Cardioprotective interventions for cancer patients receiving anthracyclines. Cochrane Database Syst Rev (6): CD003917, 2011.
-
Wexler LH, Andrich MP, Venzon D, et al.: Randomized trial of the cardioprotective agent ICRF-187 in pediatric sarcoma patients treated with doxorubicin. J Clin Oncol 14 (2): 362-72, 1996.
-
Lipshultz SE, Scully RE, Lipsitz SR, et al.: Assessment of dexrazoxane as a cardioprotectant in doxorubicin-treated children with high-risk acute lymphoblastic leukaemia: long-term follow-up of a prospective, randomised, multicentre trial. Lancet Oncol 11 (10): 950-61, 2010.
-
Asselin BL, Devidas M, Chen L, et al.: Cardioprotection and Safety of Dexrazoxane in Patients Treated for Newly Diagnosed T-Cell Acute Lymphoblastic Leukemia or Advanced-Stage Lymphoblastic Non-Hodgkin Lymphoma: A Report of the Children's Oncology Group Randomized Trial Pediatric Oncology Group 9404. J Clin Oncol 34 (8): 854-62, 2016.
-
Shaikh F, Dupuis LL, Alexander S, et al.: Cardioprotection and Second Malignant Neoplasms Associated With Dexrazoxane in Children Receiving Anthracycline Chemotherapy: A Systematic Review and Meta-Analysis. J Natl Cancer Inst 108 (4): , 2016.
-
Schwartz CL, Constine LS, Villaluna D, et al.: A risk-adapted, response-based approach using ABVE-PC for children and adolescents with intermediate- and high-risk Hodgkin lymphoma: the results of P9425. Blood 114 (10): 2051-9, 2009.
-
Chow EJ, Aplenc R, Vrooman LM, et al.: Late health outcomes after dexrazoxane treatment: A report from the Children's Oncology Group. Cancer 128 (4): 788-796, 2022.
-
Chow EJ, Aggarwal S, Doody DR, et al.: Dexrazoxane and Long-Term Heart Function in Survivors of Childhood Cancer. J Clin Oncol 41 (12): 2248-2257, 2023.
-
Mansouri I, Allodji RS, Hill C, et al.: The role of irradiated heart and left ventricular volumes in heart failure occurrence after childhood cancer. Eur J Heart Fail 21 (4): 509-518, 2019.
-
Shrestha S, Bates JE, Liu Q, et al.: Radiation therapy related cardiac disease risk in childhood cancer survivors: Updated dosimetry analysis from the Childhood Cancer Survivor Study. Radiother Oncol 163: 199-208, 2021.
-
Lo AC, Liu A, Liu Q, et al.: Late Cardiac Toxic Effects Associated With Treatment Protocols for Hodgkin Lymphoma in Children. JAMA Netw Open 7 (1): e2351062, 2024.
-
Murphy ES, Xie H, Merchant TE, et al.: Review of cranial radiotherapy-induced vasculopathy. J Neurooncol 122 (3): 421-9, 2015.
-
Passos J, Nzwalo H, Valente M, et al.: Microbleeds and cavernomas after radiotherapy for paediatric primary brain tumours. J Neurol Sci 372: 413-416, 2017.
-
Bowers DC, Liu Y, Leisenring W, et al.: Late-occurring stroke among long-term survivors of childhood leukemia and brain tumors: a report from the Childhood Cancer Survivor Study. J Clin Oncol 24 (33): 5277-82, 2006.
-
De Bruin ML, Dorresteijn LD, van't Veer MB, et al.: Increased risk of stroke and transient ischemic attack in 5-year survivors of Hodgkin lymphoma. J Natl Cancer Inst 101 (13): 928-37, 2009.
-
van Dijk IW, van der Pal HJ, van Os RM, et al.: Risk of Symptomatic Stroke After Radiation Therapy for Childhood Cancer: A Long-Term Follow-Up Cohort Analysis. Int J Radiat Oncol Biol Phys 96 (3): 597-605, 2016.
-
Reulen RC, Guha J, Bright CJ, et al.: Risk of cerebrovascular disease among 13 457 five-year survivors of childhood cancer: A population-based cohort study. Int J Cancer 148 (3): 572-583, 2021.
-
Waxer JF, Wong K, Modiri A, et al.: Risk of Cerebrovascular Events Among Childhood and Adolescent Patients Receiving Cranial Radiation Therapy: A PENTEC Normal Tissue Outcomes Comprehensive Review. Int J Radiat Oncol Biol Phys 119 (2): 417-430, 2024.
-
Mueller S, Sear K, Hills NK, et al.: Risk of first and recurrent stroke in childhood cancer survivors treated with cranial and cervical radiation therapy. Int J Radiat Oncol Biol Phys 86 (4): 643-8, 2013.
-
Fullerton HJ, Stratton K, Mueller S, et al.: Recurrent stroke in childhood cancer survivors. Neurology 85 (12): 1056-64, 2015.
-
Chow EJ, Chen Y, Hudson MM, et al.: Prediction of Ischemic Heart Disease and Stroke in Survivors of Childhood Cancer. J Clin Oncol 36 (1): 44-52, 2018.
-
El-Fayech C, Haddy N, Allodji RS, et al.: Cerebrovascular Diseases in Childhood Cancer Survivors: Role of the Radiation Dose to Willis Circle Arteries. Int J Radiat Oncol Biol Phys 97 (2): 278-286, 2017.
-
Bright CJ, Hawkins MM, Guha J, et al.: Risk of Cerebrovascular Events in 178 962 Five-Year Survivors of Cancer Diagnosed at 15 to 39 Years of Age: The TYACSS (Teenage and Young Adult Cancer Survivor Study). Circulation 135 (13): 1194-1210, 2017.
-
Mueller S, Kline CN, Buerki RA, et al.: Stroke impact on mortality and psychologic morbidity within the Childhood Cancer Survivor Study. Cancer 126 (5): 1051-1059, 2020.
-
Walker AJ, Grainge MJ, Card TR, et al.: Venous thromboembolism in children with cancer - a population-based cohort study. Thromb Res 133 (3): 340-4, 2014.
-
Madenci AL, Weil BR, Liu Q, et al.: Long-Term Risk of Venous Thromboembolism in Survivors of Childhood Cancer: A Report From the Childhood Cancer Survivor Study. J Clin Oncol : JCO2018784595, 2018.
-
Mueller S, Fullerton HJ, Stratton K, et al.: Radiation, atherosclerotic risk factors, and stroke risk in survivors of pediatric cancer: a report from the Childhood Cancer Survivor Study. Int J Radiat Oncol Biol Phys 86 (4): 649-55, 2013.
-
Chao C, Xu L, Bhatia S, et al.: Cardiovascular Disease Risk Profiles in Survivors of Adolescent and Young Adult (AYA) Cancer: The Kaiser Permanente AYA Cancer Survivors Study. J Clin Oncol 34 (14): 1626-33, 2016.
-
Winther JF, Bhatia S, Cederkvist L, et al.: Risk of cardiovascular disease among Nordic childhood cancer survivors with diabetes mellitus: A report from adult life after childhood cancer in Scandinavia. Cancer 124 (22): 4393-4400, 2018.
-
van Dalen EC, Mulder RL, Suh E, et al.: Coronary artery disease surveillance among childhood, adolescent and young adult cancer survivors: A systematic review and recommendations from the International Late Effects of Childhood Cancer Guideline Harmonization Group. Eur J Cancer 156: 127-137, 2021.
-
Lipshultz SE, Lipsitz SR, Kutok JL, et al.: Impact of hemochromatosis gene mutations on cardiac status in doxorubicin-treated survivors of childhood high-risk leukemia. Cancer 119 (19): 3555-62, 2013.
-
Visscher H, Ross CJ, Rassekh SR, et al.: Validation of variants in SLC28A3 and UGT1A6 as genetic markers predictive of anthracycline-induced cardiotoxicity in children. Pediatr Blood Cancer 60 (8): 1375-81, 2013.
-
Wang X, Liu W, Sun CL, et al.: Hyaluronan synthase 3 variant and anthracycline-related cardiomyopathy: a report from the children's oncology group. J Clin Oncol 32 (7): 647-53, 2014.
-
Wang X, Sun CL, Quiñones-Lombraña A, et al.: CELF4 Variant and Anthracycline-Related Cardiomyopathy: A Children's Oncology Group Genome-Wide Association Study. J Clin Oncol 34 (8): 863-70, 2016.
-
Aminkeng F, Bhavsar AP, Visscher H, et al.: A coding variant in RARG confers susceptibility to anthracycline-induced cardiotoxicity in childhood cancer. Nat Genet 47 (9): 1079-84, 2015.
-
Visscher H, Rassekh SR, Sandor GS, et al.: Genetic variants in SLC22A17 and SLC22A7 are associated with anthracycline-induced cardiotoxicity in children. Pharmacogenomics 16 (10): 1065-76, 2015.
-
Singh P, Wang X, Hageman L, et al.: Association of GSTM1 null variant with anthracycline-related cardiomyopathy after childhood cancer-A Children's Oncology Group ALTE03N1 report. Cancer 126 (17): 4051-4058, 2020.
-
Sapkota Y, Ehrhardt MJ, Qin N, et al.: A Novel Locus on 6p21.2 for Cancer Treatment-Induced Cardiac Dysfunction Among Childhood Cancer Survivors. J Natl Cancer Inst 114 (8): 1109-1116, 2022.
-
Davies SM: Getting to the heart of the matter. J Clin Oncol 30 (13): 1399-400, 2012.
-
Lewey J, Haythe J: Cardiomyopathy in pregnancy. Semin Perinatol 38 (5): 309-17, 2014.
-
Hines MR, Mulrooney DA, Hudson MM, et al.: Pregnancy-associated cardiomyopathy in survivors of childhood cancer. J Cancer Surviv 10 (1): 113-21, 2016.
-
Chait-Rubinek L, Mariani JA, Goroncy N, et al.: A Retrospective Evaluation of Risk of Peripartum Cardiac Dysfunction in Survivors of Childhood, Adolescent and Young Adult Malignancies. Cancers (Basel) 11 (8): , 2019.
-
Liu S, Aghel N, Belford L, et al.: Cardiac Outcomes in Pregnant Women With Treated Cancer. J Am Coll Cardiol 72 (17): 2087-2089, 2018.
-
Bottinor W, Im C, Doody DR, et al.: Mortality After Major Cardiovascular Events in Survivors of Childhood Cancer. J Am Coll Cardiol 83 (8): 827-838, 2024.
-
Dietz AC, Seidel K, Leisenring WM, et al.: Solid organ transplantation after treatment for childhood cancer: a retrospective cohort analysis from the Childhood Cancer Survivor Study. Lancet Oncol 20 (10): 1420-1431, 2019.
-
Brouwer CA, Postma A, Hooimeijer HL, et al.: Endothelial damage in long-term survivors of childhood cancer. J Clin Oncol 31 (31): 3906-13, 2013.
-
Maraldo MV, Jørgensen M, Brodin NP, et al.: The impact of involved node, involved field and mantle field radiotherapy on estimated radiation doses and risk of late effects for pediatric patients with Hodgkin lymphoma. Pediatr Blood Cancer 61 (4): 717-22, 2014.
-
Tukenova M, Guibout C, Oberlin O, et al.: Role of cancer treatment in long-term overall and cardiovascular mortality after childhood cancer. J Clin Oncol 28 (8): 1308-15, 2010.
-
Armstrong GT, Kawashima T, Leisenring W, et al.: Aging and risk of severe, disabling, life-threatening, and fatal events in the childhood cancer survivor study. J Clin Oncol 32 (12): 1218-27, 2014.
-
Chen AB, Punglia RS, Kuntz KM, et al.: Cost effectiveness and screening interval of lipid screening in Hodgkin's lymphoma survivors. J Clin Oncol 27 (32): 5383-9, 2009.
-
Wong FL, Bhatia S, Landier W, et al.: Cost-effectiveness of the children's oncology group long-term follow-up screening guidelines for childhood cancer survivors at risk for treatment-related heart failure. Ann Intern Med 160 (10): 672-83, 2014.
-
Yeh JM, Nohria A, Diller L: Routine echocardiography screening for asymptomatic left ventricular dysfunction in childhood cancer survivors: a model-based estimation of the clinical and economic effects. Ann Intern Med 160 (10): 661-71, 2014.
-
Landier W, Armenian SH, Lee J, et al.: Yield of screening for long-term complications using the children's oncology group long-term follow-up guidelines. J Clin Oncol 30 (35): 4401-8, 2012.
-
Ramjaun A, AlDuhaiby E, Ahmed S, et al.: Echocardiographic Detection of Cardiac Dysfunction in Childhood Cancer Survivors: How Long Is Screening Required? Pediatr Blood Cancer 62 (12): 2197-203, 2015.
-
Spewak MB, Williamson RS, Mertens AC, et al.: Yield of screening echocardiograms during pediatric follow-up in survivors treated with anthracyclines and cardiotoxic radiation. Pediatr Blood Cancer 64 (6): , 2017.
-
Chen Y, Chow EJ, Oeffinger KC, et al.: Traditional Cardiovascular Risk Factors and Individual Prediction of Cardiovascular Events in Childhood Cancer Survivors. J Natl Cancer Inst 112 (3): 256-265, 2020.
-
Scott JM, Li N, Liu Q, et al.: Association of Exercise With Mortality in Adult Survivors of Childhood Cancer. JAMA Oncol 4 (10): 1352-1358, 2018.
-
Schindera C, Zürcher SJ, Jung R, et al.: Physical fitness and modifiable cardiovascular disease risk factors in survivors of childhood cancer: A report from the SURfit study. Cancer 127 (10): 1690-1698, 2021.
-
Rueegg CS, Zürcher SJ, Schindera C, et al.: Effect of a 1-year physical activity intervention on cardiovascular health in long-term childhood cancer survivors-a randomised controlled trial (SURfit). Br J Cancer 129 (8): 1284-1297, 2023.
Late Effects of the Central Nervous System
Neurocognitive
Neurocognitive late effects are commonly observed after treatment of malignancies that require central nervous system (CNS)–directed therapies, including the following:
- Cranial radiation therapy.
- Systemic therapy with high-dose methotrexate or cytarabine.
- Intrathecal chemotherapy.
- Neurosurgical procedures.[1,2]
Children with CNS tumors or acute lymphoblastic leukemia (ALL) are most likely to be affected. Risk factors for the development of neurocognitive late effects include the following:[3,4,5,6,7]
- Female sex.
- Younger age at the time of treatment.
- Tumor location.
- Treatment with cranial radiation therapy and/or chemotherapeutic agents (systemic or intrathecal).
- Higher cranial radiation dose.
- Time since treatment.
Cognitive phenotypes observed in childhood survivors of ALL and CNS tumors may differ from traditional developmental disorders. For example, the phenotype of attention problems in ALL and brain tumor survivors appears to differ from developmental attention-deficit/hyperactivity disorder (ADHD) in that few survivors demonstrate significant hyperactivity/impulsivity, but instead have associated difficulties with processing speed and executive function.[8,9]
A Pediatric Normal Tissue Effects in the Clinic (PENTEC) comprehensive review was performed to develop models to facilitate the identification of dose constraints for radiation-associated CNS morbidities.[10]
- Models suggest a 5% risk of a subsequent intelligence quotient (IQ) lower than 85 when the following occurs:
- 10% of the brain is irradiated to 35.7 Gy.
- 20% of the brain is irradiated to 29.1 Gy.
- 50% of the brain is irradiated to 22.2 Gy.
- 100% of the brain is irradiated to 18.1 Gy.
- Note: All at 2 Gy/fraction and without methotrexate.
- Methotrexate exposure increased the risk of an IQ score lower than 85, similar to a generalized uniform brain radiation dose of 5.9 Gy. However, a limitation to this analysis was a lack of data about the total methotrexate doses, both intravenous and intrathecal.
- The model for predicting expected IQ, which also included the effect of dose, age, and methotrexate, suggested that each of these factors has an independent but probably cumulative effect on IQ.
In addition to the direct effects of neurotoxic therapies like cranial radiation, Childhood Cancer Survivor Study (CCSS) investigators observed that chronic health conditions resulting from non-neurotoxic treatment exposures (e.g., thoracic radiation) can adversely impact neurocognitive function presumably mediated by chronic cardiopulmonary and endocrine dysfunction.[11] In addition, some sequelae of neurotoxic therapy (e.g., severe hearing loss) have been associated with neurocognitive deficits independent of the neurotoxic treatment received.[12]
A related investigation from the CCSS evaluated longitudinal associations between physical activity and neurocognitive problems in adult survivors of childhood cancer.[13]
- Survivors were less likely than their siblings to report consistent physical activity (28.1% vs. 33.6%).
- Survivors who reported more consistent physical activity had fewer neurocognitive problems and larger improvements in cognitive concerns years after treatment.
- Body mass index (BMI) and severe chronic health conditions partially mediated the physical activity–neurocognitive associations, but the mediation effects were small.
A subsequent systematic review and meta-analysis compared the effects of physical activity or exercise interventions on cognitive function among individuals diagnosed with cancer (aged 0–19 years) with that of controls. Twenty-two unique studies (16 randomized controlled trials) were found with data on 12,767 individuals.[14] The median age at the start of the study was 12 years (interquartile range [IQR], 11–14 years), the median time from the end of cancer treatment was 2.5 years (IQR, 1.1–3.0 years), and the median intervention period was 12 weeks (IQR, 10–24 weeks).
- Compared with controls, there was moderate-quality evidence that physical activity and exercise improved cognitive performance measures and patient-reported measures of cognitive function in cancer survivors.
Childhood cancer survivors may be at risk for cognitive decline throughout their lives (even if not present in the first 10 years after therapy). In a study of 2,375 adult survivors of childhood ALL, Hodgkin lymphoma, or CNS tumors (mean age at evaluation, 31.8 years) and their sibling controls, new onset memory impairment emerged more often in survivors, decades after cancer diagnosis and treatment.[15]
- Compared with siblings, a higher proportion of survivors with no impairment in memory at baseline had new-onset memory impairment at follow-up, as follows:
- Sibling proportion, 7.8%.
- ALL survivors treated with chemotherapy only, 14.0%.
- ALL survivors treated with cranial radiation therapy, 25.8%.
- CNS tumor survivors, 34.7%.
- Hodgkin lymphoma survivors, 16.6%.
- Factors associated with new-onset memory impairment included cranial radiation in survivors of CNS tumor (relative risk [RR], 1.97) and dose of 8,000 mg/m2 or more of alkylator chemotherapy in survivors of ALL treated without cranial radiation therapy (RR, 2.80).
- Neurological conditions mediated the impact of cranial radiation therapy on new-onset memory impairment in survivors of CNS tumors.
- Smoking, obesity, low education attainment, and low physical activity were associated with an elevated risk of new onset-memory impairment.
Neurocognitive outcomes in brain tumor survivors
Long-term cognitive effects caused by illness and associated treatments are well-established morbidities in survivors of childhood and adolescent brain tumors. Risk factors for adverse neurocognitive effects in this group include the following:
- Cranial radiation therapy.
- Cranial radiation therapy has been associated with the highest risk of long-term cognitive morbidity, particularly in younger children.[3,4]
- There is an established dose-response relationship, with patients who receive higher-dose cranial radiation therapy consistently performing more poorly on intellectual measures.[3,4]
- Radiation dose to specific regions of the brain, including the temporal lobes and hippocampi, have been shown to significantly impact longitudinal IQ scores and academic achievement scores among children treated with craniospinal irradiation for medulloblastoma.[16]
- Tumor site.[3]
- Shunted hydrocephalus.[17,18]
- Postsurgical cerebellar mutism.[19]
- Auditory difficulties, including sensorineural hearing loss.[12,17]
- History of stroke.[20]
- Seizures.[3,21]
- Socioeconomic status (SES).[22]
The negative impact of radiation treatment has been characterized by changes in IQ scores, which have been noted to drop about 2 to 5 years after diagnosis.[23,24,25]
- The decline continues 5 to 10 years afterward, although less is known about potential stabilization or further decline of IQ scores several decades after diagnosis.[23,25]
- The decline in IQ scores over time typically reflects the child's failure to acquire new abilities or information at a rate similar to that of his or her peers, rather than a progressive loss of skills and knowledge.[26]
- Affected children also may experience deficits in other cognitive areas, including academic domains (reading and math) and problems with attention, processing speed, memory, and visual or perceptual motor skills.[27]
- Changes in cognitive functioning may be partially explained by radiation-induced reduction of normal-appearing white matter volume or integrity of white matter pathways, as evaluated through magnetic resonance imaging (MRI).[28,29]
- Reduced white matter integrity has been directly linked to slowed cognitive processing speed in survivors of brain tumors,[30] while greater white matter volume has been associated with better working memory, particularly in females.[29]
- Data from contemporary protocols show that using lower doses of cranial radiation, proton beam radiation, and more targeted treatment volumes appears to reduce the severity of neurocognitive effects of therapy.[3,18]
Evidence (predictors of cognitive decline among survivors of CNS tumors):
Longitudinal cohort studies have provided insight into the trajectory and predictors of cognitive decline among survivors of CNS tumors.
- A multisite, prospective, longitudinal trial evaluated predictors of cognitive performance among 139 infants with brain tumors who were treated with chemotherapy, with or without focal proton or photon radiation therapy.[31]
- IQ, parent-reported working memory, and parent-reported adaptive functioning were worse than normative expectations at baseline, with younger age and lower SES representing predictive factors.
- IQ remained stable over time, whereas parent-reported attention and executive dysfunction increased.
- Cognitive outcomes did not differ by treatment exposure (chemotherapy only vs. chemotherapy with radiation therapy).
- Changes in cognitive function were associated with supratentorial tumor location and cerebrospinal fluid diversion.
- St. Jude Children's Research Hospital (SJCRH) studied 78 children younger than 20 years (mean, 9.7 years) diagnosed with a low-grade glioma.[32]
- Cognitive decline after 54 Gy of conformal cranial radiation therapy was noted (see Figure 5).
- Age at time of cranial irradiation was more important than was cranial radiation dose in predicting cognitive decline, with children younger than 5 years estimated to experience the greatest cognitive decline.
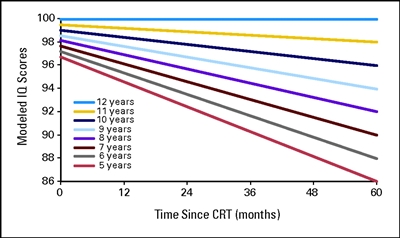
Figure 5. Modeled intelligence quotient (IQ) scores after conformal radiation therapy (CRT) by age for pediatric low-grade glioma. Age is measured in years, and time is measured in months after the start of CRT. Thomas E. Merchant, Heather M. Conklin, Shengjie Wu, Robert H. Lustig, and Xiaoping Xiong, Late Effects of Conformal Radiation Therapy for Pediatric Patients With Low-Grade Glioma: Prospective Evaluation of Cognitive, Endocrine, and Hearing Deficits, Journal of Clinical Oncology, volume 27, issue 22, pages 3691-3697. Reprinted with permission. © (2009) American Society of Clinical Oncology. All rights reserved.
- In a study of 51 children with low-grade gliomas and low-grade glioneural tumors diagnosed within the first year of life, the following was reported:[33]
- Mean IQ score was 75.5; 75% of the children had IQ scores lower than 85.
- Predictors of low IQ included a supratentorial location of the primary tumor and treatment with more chemotherapy regimens but not radiation use.
- The child's ability to complete age-appropriate tasks was as affected as IQ scores.
- A study of 126 medulloblastoma survivors treated with 23.4 Gy or 36 Gy to 39.6 Gy of craniospinal radiation (with a conformal boost dose of 55.8 Gy to the primary tumor bed) assessed processing speed, attention, and memory performance.[34]
- Processing speed scores declined significantly over time, while less decline was observed in attention and memory performance. Higher doses of radiation and younger age at diagnosis predicted slower processing speed over time.
- Studies of working memory and academic achievement in patients enrolled on the same medulloblastoma trial (SJCRH SJMB03 [NCT00085202]) indicated that performance was largely within the age-expected range up to 5 years postdiagnosis,[35,36] although in both studies, posterior fossa syndrome, higher cranial radiation dose, and younger age at diagnosis predicted worse performance over time.
- Serious hearing loss was associated with intellectual and academic decline over time.[36]
- In a prospective study of 178 children with medulloblastoma, 60 (34%) developed posterior fossa syndrome. Of these patients, 40 (23%) developed complete mutism and 20 (11%) developed diminished speech.[37]
- All children with posterior fossa syndrome had severe ataxia, and 42.5% of patients with posterior fossa syndrome and complete mutism had movement disorders.
- Independent risk factors for posterior fossa syndrome included younger age and surgery in a low-volume surgery center. Risk was lower among children with sonic hedgehog (SHH) tumors.
- Speech and gait returned in children with posterior fossa syndrome and complete mutism at a median of 2.3 and 0.7 months, respectively. Speech and gait returned in children with posterior fossa syndrome and diminished speech at a median of 2.1 and 1.5 months, respectively. However, with 12 months of follow-up, 12 of 27 children (44.4%) with posterior fossa syndrome and complete mutism were nonambulatory at 1 year.
- The presence of a movement disorder or high ataxia score was associated with delayed speech recovery, whereas older age and high ataxia score were associated with delayed gait return.
- Symptoms improved in all children, but no child with posterior fossa syndrome had a normal neurological examination at a median of 23 months after surgery.
- A prospective study compared 36 pediatric patients with medulloblastoma who experienced posterior fossa syndrome with 36 patients with medulloblastoma who did not experience posterior fossa syndrome but were matched on treatment and age at diagnosis.[37,38]
- The posterior fossa syndrome group demonstrated lower mean scores at 1, 3, and 5 years postdiagnosis on general intellectual ability, processing speed, working memory, and spatial relations compared with the non–posterior fossa syndrome group.
- The group who experienced posterior fossa syndrome showed little recovery over time and further decline over time in some domains (attention and working memory), compared with the non–posterior fossa syndrome group.
- Canadian investigators evaluated the impact of radiation (dose and boost volume) and neurological complications on patterns of intellectual functioning in a cohort of 113 medulloblastoma survivors (mean age at diagnosis, 7.5 years; mean time from diagnosis to last assessment, 6 years).[4]
- Survivors treated with reduced-dose craniospinal radiation therapy plus tumor bed boost showed stable intellectual functioning.
- Neurological complications, such as hydrocephalus requiring cerebrospinal fluid diversion and mutism, and treatment with higher doses and larger boost volumes of radiation resulted in intellectual declines with distinctive trajectories.
- Studies are beginning to examine cognitive outcomes in histologically distinct subtypes of brain tumors.
- Data from a sample of 121 medulloblastoma patients demonstrated variation in cognitive outcomes by four distinct molecular subgroups and differences in patterns of change over time.[39]
- Future research is required to establish if neurocognitive outcomes vary across biologically distinct subtypes of childhood brain tumors.
- SES has been shown to predict long-term cognitive outcomes in brain tumor survivors. In a prospective, longitudinal, phase II study of 248 children who were treated with conformal radiation therapy (54–59.4 Gy) for ependymoma, low-grade glioma, or craniopharyngioma, patients were monitored serially with cognitive assessment for 10 years.[22]
- At preradiation therapy baseline, significant associations were seen between SES and IQ, reading and math scores, sustained attention, and adaptive function. Higher SES was associated with better performance (P < .005).
- SES predicted change over time in IQ and reading and math scores. Higher SES was associated with less decline (P < .001).
- These results suggest that higher SES is a protective factor for cognitive late effects.
Evidence (predictors of cognitive decline among long-term survivors of CNS tumors):
Although adverse neurocognitive outcomes observed 5 to 10 years after treatment are presumed to be pervasive, and potentially worsen over time, few empirical data are available regarding the neurocognitive functioning in very long-term survivors of CNS tumors.
- A longitudinal study evaluated the relationship of hippocampal dose and short-term memory decline in 80 children and adolescents with low-grade gliomas (median age, 9.5 years at treatment) who received 54 Gy of radiation therapy.[40]
- At a median neurocognitive follow-up of 9.8 years, higher hippocampal dose (volume receiving 40 Gy) was associated with a greater decline in delayed recall.
- Among adult survivors participating in the CCSS, CNS tumor survivors (n = 802) self-reported significantly more problems with attention/processing speed, memory, emotional control, and organization than did survivors of non-CNS malignancies (n = 5,937) and sibling controls (n = 382).[41]
- Another CCSS study evaluating patterns of late mortality and morbidity in 2,821 adult survivors of CNS tumors reported impairment on measures of attention/processing speed (42.9%–73.3%) and memory (14.3%–37.4%), with differences observed by diagnosis and cranial radiation dose.[42]
- A study of 224 adult survivors of pediatric brain tumors participating in the St. Jude Life (SJLIFE) cohort study revealed that 20% to 30% of the survivors demonstrated severe neurocognitive impairment (defined as at least two standard deviations below normative mean) on clinical assessments of intelligence, memory, and executive function (e.g., planning, organization, and flexibility).[3]
- Among adults in the general population, the expected impairment rate at this threshold is 2%.
- Survivors who received whole-brain cranial irradiation were 1.5 to 3 times more likely to have severe neurocognitive impairment than were survivors who did not receive any cranial irradiation.
- Hydrocephalus with shunt placement and seizures were also associated with increased risk of impairment.
- In the CCSS, investigators compared long-term neuropsychological and SES outcomes of 181 adult survivors of pediatric low-grade gliomas with the outcomes of an age-matched and sex-matched sibling comparison group.[43]
- Survivors who were treated with surgery and radiation therapy (median age at diagnosis, 7 years; median age at assessment, 41 years) scored lower on estimated IQ than did survivors who were treated with surgery only, who scored lower than siblings (surgery and radiation therapy, 93.9; surgery only, 101.2; siblings, 108.5; all P values < .0001).
- Younger age at diagnosis was predictive of low scores for all neuropsychological outcomes except for attention/processing speed.
- Survivors who were treated with surgery and radiation therapy had more-than-twofold–lower occupation scores, income, and education than did survivors who were treated with surgery only.
- In a retrospective review of 528 brain tumor survivors diagnosed between 2000 and 2015, the prevalence of a clinical diagnosis of ADHD was 13.1%.[44]
- Of the survivors, 12.1% used medications for ADHD, and 19.9% of survivors had symptoms of ADHD without a clinical diagnosis.
- ADHD diagnosis was associated with younger age at tumor diagnosis and supratentorial tumor location, but not with sex, tumor type, or treatment type.
The neurocognitive consequences of CNS disease and treatment may have a considerable impact on functional outcomes for brain tumor survivors.
- In childhood and adolescence, neurocognitive deficits have been associated with poor social adjustment, including problems with peer relations, social withdrawal, and reduced social skills.[45,46]
- CNS tumor survivors are more likely to need special education services than are survivors of other malignancies.[47]
- Adult CNS tumor survivors are less likely to live independently, marry, and graduate from college than are survivors of other malignancies and siblings.[48]
Cognitive outcomes after proton radiation therapy
Data are emerging regarding cognitive outcomes after proton radiation to the CNS.[49,50,51] However, these studies have been limited by retrospective analysis of cognitive outcomes among relatively small clinically heterogenous pediatric brain tumor cohorts and the use of historically treated photon patients or population standards as comparison groups.
- In studies largely describing IQ changes during early follow-up (<5 years from radiation), results demonstrate lack of difference in slopes of IQ change among photon-treated and proton-treated patients [49] and significant declines in cognitive processing speed among patients treated with proton radiation.[50]
- One study compared the intellectual trajectories between pediatric patients with medulloblastoma who were treated with proton and photon radiation therapy (4.3-year mean follow-up after median 23.4-Gy craniospinal irradiation dose). Notably, boost dose and margins were significantly different between the two groups.[51]
- Children treated with proton radiation therapy exhibited superior long-term outcomes in global IQ, perceptual reasoning, and working memory compared with children who were treated with photon radiation therapy.
- The photon radiation therapy group exhibited a significant decline in global IQ, working memory, and processing speed.
- The proton radiation therapy group exhibited stable scores over time in all domains, except for processing speed.
Considering the relatively brief follow-up time from radiation, longitudinal follow-up is important to determine whether proton radiation provides a clinically meaningful benefit in sparing cognitive function compared with photon radiation. In addition, more targeted radiation treatment volumes with photons may diminish potential differences.
Neurocognitive outcomes in acute lymphoblastic leukemia (ALL) survivors
To minimize the risk of late cognitive sequelae, contemporary therapy for ALL uses a risk-stratified approach that reserves cranial irradiation for children who are considered at high risk of CNS relapse.
ALL and cranial radiation
In survivors of ALL, cranial radiation therapy may result in clinical and radiographic neurological late sequelae, including the following:
-
Clinical leukoencephalopathy. Clinical leukoencephalopathy characterized by fluctuating encephalopathy, stroke-like symptoms, spasticity, ataxia, dysarthria, dysphagia, hemiparesis, and seizures is uncommon after contemporary ALL therapy. Neuroimaging frequently demonstrates white matter abnormalities among survivors treated with cranial irradiation and/or high-dose methotrexate.
- Radiographic leukoencephalopathy has been reported in up to 80% of children on some treatment regimens.
- Higher doses and more courses of intravenous methotrexate have been reported to increase risk of leukoencephalopathy.[52]
- In many patients, white matter anomalies are transient and decrease in prevalence, extent, and intensity with longer elapsed time from completion of therapy.[52]
- Leukoencephalopathy results in smaller white matter volumes that have been correlated with cognitive deficits.[52]
- Although these abnormalities are mild among the irradiated patients (overall IQ decline of approximately 10 points), those who have received higher doses at a young age may have significant learning difficulties.[53,54]
-
Neurocognitive deficits. Deficits in neurocognitive functions such as visual-motor integration, processing speed, attention, and short-term memory are reported in children treated with 18 Gy to 24 Gy.[53,55,56]
- Females and children treated at a younger age are more vulnerable to the adverse impact of cranial radiation on the developing brain.[5]
- The decline in intellectual functioning appears to be progressive, showing more impairment of cognitive function with increasing time since radiation therapy.[5,6]
- Limited studies suggest that long-term survivors of childhood ALL treated with cranial irradiation are at risk of progressive decline consistent with early-onset mild cognitive impairment; this risk is most prominent among those treated with cranial radiation doses of 24 Gy.[57,58]
ALL and chemotherapy-only CNS therapy
Because of its penetrance into the CNS, systemic methotrexate has been used in a variety of low-dose and high-dose regimens for leukemia CNS prophylaxis.
- Systemic methotrexate in high doses with or without radiation therapy can lead to an infrequent but well-described leukoencephalopathy, which has been linked to neurocognitive impairment.[52]
- When neurocognitive outcomes after radiation therapy and chemotherapy-only regimens are directly compared, the evidence suggests a better outcome for those treated with chemotherapy alone, although some studies show no significant difference.[59,60]
- In a longitudinal analysis of 210 childhood ALL survivors, the development of acute leukoencephalopathy during chemotherapy-only CNS therapy predicted higher risks of developing long-term neurobehavioral problems (e.g., deficits in organization and task initiation [components of executive function]) and reduced white matter integrity in frontal brain regions.[61]
- Compared with cranial irradiation, chemotherapy-only CNS-directed treatment produces neurocognitive deficits involving processes of attention, speed of information processing, memory, verbal comprehension, visual-spatial skills, visual-motor functioning, and executive functioning. Global intellectual function is typically preserved.[55,59,62,63,64]
- Few longitudinal studies evaluating long-term neurocognitive outcome report adequate data for a decline in global IQ after treatment with chemotherapy alone.[62]
- The academic achievement of ALL survivors in the long term seems to be generally average for reading and spelling, with deficits mainly affecting arithmetic performance.[59,65]
- Risk factors for poor neurocognitive outcome after chemotherapy-only CNS-directed treatment are younger age and female sex.[64,66]
- Reduced cognitive status has been observed in association with reduced integrity in neuroanatomical regions essential in memory formation (e.g., reduced hippocampal volume with increased activation and thinner parietal cortices).[61]
- The long-term impact of these prevalent neurocognitive and neuroimaging abnormalities on functional status in aging adults treated for childhood ALL, particularly those treated with contemporary approaches using chemotherapy alone, remains an active area of research.
- Research in childhood ALL survivors treated with chemotherapy alone suggests that genetic predisposition may modulate risk of developing neurocognitive late effects. It also provides insights into potential mechanisms underlying neurocognitive deficits.[67,68]
Evidence (neurocognitive functioning in large pediatric cancer survivor cohorts):
- CCSS investigators identified sex-specific associations between treatment and chronic health conditions with neurocognitive impairment among 1,207 survivors of ALL (mean age, 30.6 years) who were treated with chemotherapy only.[7]
- Both male and female survivors reported increased prevalence of impaired task efficiency (attention and processing speed; adjusted odds ratio [OR], 1.89 for males; OR, 1.50 for females) and impaired memory (OR, 1.89 for males; OR, 1.96 for females) compared with 2,273 same-sex siblings.
- Among male survivors, impaired task efficiency was associated with grades 2 to 4 neurological conditions (OR, 4.33) and pulmonary conditions (OR, 4.99). Impaired memory was associated with increased cumulative dose of intrathecal methotrexate (OR, 1.68) and exposure to dexamethasone (OR, 2.44).
- In female survivors, grades 2 to 4 endocrine conditions were associated with higher risk of impaired task efficiency (OR, 2.19) and memory (OR, 2.26).
- The CCSS examined parent-reported cognitive, behavior, and learning problems from 1,560 adolescent survivors of childhood ALL who were treated with chemotherapy alone between 1970 and 1999.[69]
- Survivors treated with cranial irradiation had significantly higher frequency of problems in anxiety-depression, inattention-hyperactivity, and social withdrawal than did patients who were not treated with cranial irradiation.
- Compared with siblings, survivors treated with chemotherapy only were more likely to demonstrate headstrong behavior (19% of survivors vs. 14% of siblings, P = .010), inattention-hyperactivity (19% vs. 14%, P < .0001), social withdrawal (18% vs. 12%, P = .002), and had higher rates of learning problems (28% vs. 14%, P < .0001).
- In multivariable models among survivors, increased cumulative dose of intravenous methotrexate (i.e., >4.3 g/m2) conferred increased risk of inattention-hyperactivity (RR, 1.53).
- Adolescent survivors with cognitive or behavior problems and those with learning problems were less likely to graduate from college as young adults than adolescent survivors without cognitive or behavior problems.
- Inattention and hyperactivity problems were associated with the highest risk of special education placement during adolescence. Participation in special education during adolescence did not improve adult educational attainment.
- The SJCRH TOTAL XVI (NCT00549848) trial evaluated whether a higher dose of pegaspargase and early intensification of triple intrathecal therapy (ITT; methotrexate, hydrocortisone, and cytarabine) would improve systemic and CNS control in children with newly diagnosed ALL. Intensified ITT was defined as more than 21 cumulative doses for patients with low-risk ALL (70 of 194) and more than 27 doses for those with standard- and high-risk ALL (81 of 206). Neurocognitive testing was completed at the end of therapy.[70]
- The overall group had below age-based norms on measures of global intelligence (P < .0001), attention (P = .0051), working memory (P = .0001), processing speed (P = .0002), fine motor speed (P = .0001), and math skills (P = .0087). Caregiver ratings of patient functioning showed elevated risks of problems in attention (P = .0173), executive function (P = .0001), and adaptive skills (P = .0001).
- In the low-risk group, there were no significant differences between patients treated with and without intensified ITT.
- Patients with standard- and high-risk ALL who were treated with intensified ITT had poorer working memory (P = .0328) and fine motor speed (P = .0403), and elevated ratings of inattention (P = .0189) and executive dysfunction (P = .0245). Females in this risk group treated with intensified ITT had lower working memory scores.
- In the SJCRH Total XV (NCT00137111) trial, which omitted prophylactic cranial irradiation, comprehensive cognitive testing of 243 participants at week 120 revealed the following:[71]
- A higher risk of below-average performance on a measure of sustained attention but not on measures of intellectual functioning, academic skills, or memory.
- The risk of cognitive deficits correlated with treatment intensity but not with age at diagnosis or sex.
- Prolonged follow-up (average, 7.7 years from diagnosis) of this cohort demonstrated that intelligence was within normal limits compared with population expectations, but measures of executive function, processing speed, and memory were less than population means.
- Higher plasma methotrexate was associated with executive dysfunction, thicker cerebral cortex, and higher activity in frontal brain regions on functional MRI.
- In a large prospective study of neurocognitive outcomes in children with newly diagnosed ALL, 555 children were randomly assigned to receive CNS-directed therapy according to risk group.[72]
- The low-risk group was randomly assigned to receive either intrathecal methotrexate or high-dose methotrexate.
- The high-risk group was randomly assigned to receive either high-dose methotrexate or 24 Gy of cranial radiation therapy.
- A significant reduction in IQ scores (4–7 points) was observed in all patient groups when compared with controls, regardless of the CNS treatment delivered.
- Children younger than 5 years at diagnosis were more likely to have IQs below 80 at 3 years posttherapy than were children older than 5 years at diagnosis, irrespective of treatment allocation, suggesting that younger children are more vulnerable to treatment-related neurological toxic effects.
- Persistent cognitive deficits and progressive intellectual decline have been observed in cohorts of adults treated for ALL during childhood and associated with reduced educational attainment and unemployment.[5,54,58] The results of a study of more than 500 adult survivors of childhood ALL (average, 26 years postdiagnosis) showed the following:[54]
- Survivors demonstrated increased rates of impairment across all neurocognitive domains (ranging from 28.6%–58.9% for each domain).
- Rate of severe impairment increased as a function of cranial radiation dose and was common among survivors treated with lower doses of cranial irradiation and chemotherapy only.
- Impairment in executive function skills increased with time since diagnosis in a cranial radiation dose-dependent manner; impairment in intellect, academics, and memory progressively increased with younger age at treatment in a cranial radiation dose-dependent manner; and neurocognitive impairment was related to functional outcomes as adults, including reduced likelihood of college graduation and full-time employment.
ALL and steroid therapy
The type of steroid used for ALL systemic treatment may affect cognitive functioning.
- In a study that involved long-term neurocognitive testing (mean follow-up, 9.8 years) of 92 children with a history of standard-risk ALL who had received either dexamethasone or prednisone during treatment, no meaningful differences in mean neurocognitive and academic performance scores were observed.[73]
- In contrast, in a study of 567 adult survivors of childhood leukemia (mean age, 33 years; mean time since diagnosis, 26 years), the following was reported:[54]
- Dexamethasone exposure was associated with increased risk of impairment in attention (RR, 2.12; 95% confidence interval [CI], 1.11–4.03) and executive function (RR, 2.42; 95% CI, 1.20–4.91), independent of methotrexate exposure.
- Intrathecal hydrocortisone also increased risk of attention problems (RR, 1.24; 95% CI, 1.05–1.46).
Other cancers
Neurocognitive abnormalities have been reported in other groups of cancer survivors. A study of adult survivors of childhood non-CNS cancers (including ALL, n = 5,937) reported the following:[56]
- 13% to 21% of survivors reported impairment in task efficiency, organization, memory, or emotional regulation. This rate of impairment was approximately 50% higher than that reported in the sibling comparison group.
- Factors such as diagnosis before age 6 years, female sex, cranial radiation therapy, and hearing impediment were associated with impairment.
Emerging data suggest that the development of chronic health conditions in adulthood may contribute to cognitive deficits in long-term survivors of non-CNS cancers.
An SJLIFE cohort study evaluated whether children who experienced CNS injury were at higher risk of neurocognitive impairment associated with subsequent late-onset chronic health conditions. A total of 2,859 survivors who were aged 18 years or older and at least 10 years from diagnosis completed a neurocognitive battery and clinical examination. Of these patients, 1,598 had received CNS-directed therapy, including cranial radiation, intrathecal methotrexate, or neurosurgery.[74]
- CNS-treated survivors performed worse than non–CNS-treated survivors on all neurocognitive tests, and they were more likely to have global neurocognitive impairment (46.9% vs. 35.3%).
- A dose-response association was observed between severity/burden score of chronic health conditions and global neurocognitive impairment only among CNS-treated survivors (high OR, 2.24; 95% CI, 1.42–3.53; very high OR, 4.07; 95% CI, 2.30–7.17).
- Cardiovascular and pulmonary conditions were associated with cognitive outcomes (processing speed, executive function, and memory impairment) only in CNS-treated survivors with neurological conditions.
Neurocognitive abnormalities have been reported for the following cancers:
-
Acute myeloid leukemia (AML). CCSS investigators compared the neurocognitive and psychosocial outcomes of 482 survivors (median age, 30 years) with those of 3,190 sibling controls (median age, 32 years).[75]
- Compared with siblings, AML survivors were more likely to report impairment in overall emotional (RR, 2.19; 95% CI, 1.51–3.18), neurocognitive (RR, 2.03; 95% CI, 1.47–2.79), and physical quality of life (RR, 2.71; 95% CI, 1.61–4.56) outcomes.
- Survivors were also at increased risk of psychosocial deficits, including lower educational attainment (RR, 1.15; 95% CI, 1.03–1.30), unemployment (RR, 1.41; 95% CI, 1.16–1.71), and lower income (RR, 1.39; 95% CI, 1.17–1.65).
- Outcomes were not statistically different between survivors who were treated with transplant and survivors who were treated with intensive chemotherapy alone.
-
Osteosarcoma. In a study evaluating neurocognitive function among 80 long-term survivors of osteosarcoma (mean time since diagnosis, 24.7 years), survivors demonstrated lower mean scores in reading skills, attention, memory, and processing speed than did community controls.[76]
- The presence of cardiac, pulmonary, and endocrine conditions were significantly associated with worse performance on measures of memory and processing speed.
-
Soft tissue sarcoma. SJLIFE study investigators evaluated neurocognitive function and health status through objective clinical assessments in 150 survivors of childhood soft tissue sarcoma (median age, 33 years; median time from diagnosis, 24 years).[77]
- Compared with community and population controls, survivors demonstrated lower measures of verbal reasoning, mathematics, and long-term memory.
- Cumulative anthracycline exposure (per 100 mg/m2) was found to be associated with poorer verbal reasoning, reading, and patient-reported vitality.
- Neurological and neurosensory chronic conditions were associated with poorer mathematics scores and hearing impairment.
- Better cognitive performance was associated with higher social attainment.
-
Wilms tumor. SJLIFE study investigators examined the prevalence and predictors of neurocognitive outcomes, social attainment, emotional distress, and health-related quality of life in long-term survivors of pediatric Wilms tumor (n = 158; median time since diagnosis, 29 years; median age, 33 years) and compared the results to community controls.[78]
- Long-term Wilms tumor survivors demonstrated lower neurocognitive function, including verbal reasoning, academics, attention, memory, and executive function, than did controls.
- Wilms tumor survivors were less likely to graduate from college (OR, 2.23) and had more moderate-to-severe neurological conditions than did controls (18.4% vs. 8.2%; P < .001).
-
Retinoblastoma. Serial assessment of cognitive and adaptive functioning in a group of retinoblastoma survivors younger than 6 years revealed declines in developmental functioning over time, with the most pronounced declines observed in patients with 13q deletions.[79]
- Further longitudinal follow-up of this cohort identified improvement in adaptive functioning in all treatment groups and in cognitive function in survivors who were treated with enucleation alone from the age of 5 years to the age of 10 years.[80]
- At age 10 years, overall functioning was generally within the average range, although estimated IQ was significantly below the normative mean for children who were treated with enucleation alone.[80]
A study of very long-term adult survivors, who were on average 33 years postdiagnosis, demonstrated largely average cognitive functioning across domains of intelligence, memory, attention, and executive function.[81]
-
Lymphoma. Survivors of lymphoma have not historically been considered at risk of developing neurocognitive late effects.
- However, one report observed that more than two-thirds of survivors of childhood non-Hodgkin lymphoma experienced at least mild neurocognitive impairment, including severe deficits in executive function (13%), attention (9%), and memory (4%).[82]
- Similarly, in a study of 62 adult survivors of childhood Hodgkin lymphoma, survivors demonstrated worse performance on measures of sustained attention, short- and long-term memory, and cognitive fluency when compared with national normative data.[83] Importantly, measures of cardiac and pulmonary function were also associated with neurocognitive impairment in this group of survivors.
-
Neuroblastoma. CCSS investigators compared cognitive outcomes among 837 survivors of neuroblastoma (median age, 1 year at diagnosis; median attained age, 25 years) and a sibling cohort (median attained age, 23 years) using the CCSS Neurocognitive Questionnaire (NCQ).[84]
- Survivors had a 50% higher risk of impairment with attention/processing speed and emotional regulation.
- The risk of neurocognitive impairments was higher among survivors with chronic health conditions.
- Survivors were also less likely to attain adult milestones, such as living independently.
Hematopoietic stem cell transplant (HSCT)
Cognitive and academic consequences of HSCT in children have also been evaluated and include, but are not limited to, the following:
- A survey study evaluated late neurocognitive outcomes among 199 survivors of HSCT (HSCT at age <21 years; median follow-up from HSCT, 27.6 years).[85]
- As assessed by the CCSS NCQ, 18.9% to 32.5% of survivors reported impairments in task efficiency, memory, emotional regulation, or organization, compared with the expected rate of 10% (general population).
- Quality of life, as assessed by Neuro-Quality of Life Cognitive Function Short Form (Neuro-QoL), among survivors (average T score, 49.6) was comparable to that of the normative population (50).
- Independent risk factors for impaired Neuro-QoL (T score <40) included hearing issues (OR, 4.7; 95% CI, 1.96–12.6), history of stroke or seizure (OR, 4.46; 95% CI, 1.44–13.8), and sleep disturbances (OR, 6.96; 95% CI, 2.53–19.1).
- SJCRH investigators examined the influence of age and conditioning with total-body irradiation (TBI) on the trajectory of cognitive function after HSCT. Among 315 patients who completed a baseline assessment, 183 were alive at 1 year after HSCT and completed additional assessments at 1, 3, and 5 years.[86]
- At 5 years after HSCT, younger patients (aged <3 years at baseline) who received TBI demonstrated a significantly lower IQ than those who did not receive TBI.
- Longitudinal analyses demonstrated a significant impact of age and TBI over time with declines in cognitive functioning during the first year among the youngest patients and recovery of functioning in subsequent years among patients who did not receive TBI.
- Young patients who received TBI failed to recover the losses experienced during the first year after HSCT, demonstrating stability in their functioning, but at a lower level.
Neurological Sequelae
Risk of neurological complications may be predisposed by the following:
- Tumor location.
- Neurosurgical procedures.
- Cranial radiation therapy.
- Spinal radiation therapy.
- Specific neurotoxic chemotherapeutic agents.
In children with CNS tumors, mass effect, tumor infiltration, and increased intracranial pressure may result in motor or sensory deficits, cerebellar dysfunction, and secondary effects such as seizures and cerebrovascular complications.[87]
Numerous reports describe abnormalities of CNS integrity and function, but such studies are typically limited by small sample size, cohort selection and participation bias, cross-sectional ascertainment of outcomes, and variable time of assessment from treatment exposures. In contrast, relatively few studies comprehensively or systematically ascertain outcomes related to peripheral nervous system function.
CNS tumor survivors remain at higher risk of new-onset adverse neurological events across their lifetimes than siblings. No plateau has been reached for new adverse sequelae, even 30 years from diagnosis, according to a longitudinal study of 1,876 5-year survivors of CNS tumors from the CCSS. The median time from diagnosis was 23 years, and the median age of the patients studied was 30.3 years.[88]
- Cranial radiation, stroke, tumor recurrence, and development of meningioma were independently associated with late-onset neurological sequelae (seizures, focal neurological dysfunction, and neurosensory abnormalities).
- This finding supports the need to monitor these patients carefully with continued neurological follow-up within or in close association with a multidisciplinary cancer survivor clinic.
Neurological complications that may occur in survivors of childhood cancer include the following:
Seizures
The development of seizures may occur secondary to tumor mass effect within the CNS and/or from neurotoxic CNS-directed therapies.
- In 1,876 5-year survivors of CNS tumors from the CCSS, the incidence of seizures increased from 27% in survivors 5 years from diagnosis to 41% in survivors 30 years from diagnosis.[88]
- Late-onset seizures were associated with frontal lobe radiation of 50 Gy (hazard ratio [HR], 1.8) and temporal lobe radiation in a dose-dependent fashion (HR, 1.9 for 1–49 Gy; HR, 2.2 for >50 Gy).
- Other risk factors associated with late-onset seizures included recurrence (HR, 2.3), development of meningioma (HR, 2.6), and history of stroke (HR, 2.0).
- The risk of seizures was elevated for survivors compared with siblings (HR, 12.7).
- Among survivors of childhood leukemia in the CCSS (N = 4,151; 64.5% treated with cranial irradiation), 6.1% reported the development of a seizure disorder, and seizures occurred more than 5 years after diagnosis in 51% of these patients.[89]
- An SJLIFE study evaluated the impact of seizure-related factors on neurocognitive, health-related quality of life (HRQOL), and social outcomes in 2,022 childhood cancer survivors (median age, 31.5 years; median time from diagnosis, 23.6 years).[90]
- Seizures were identified in 232 survivors (11.5%; 29.9% of survivors with CNS tumors and 9.0% of survivors without CNS tumors).
- Among CNS tumor survivors, seizures were associated with poorer (based on expected age-adjusted standard z-scores) executive function and processing speed. Seizure resolution was associated with improved attention and memory.
- Among non-CNS tumor survivors, seizures were associated with worse function in all cognitive domains compared with survivors without seizures. Seizure severity was associated with worse processing speed, and resolution of seizures was associated with better executive function and attention.
- Non-CNS survivors with seizures had poorer HRQOL outcomes (physical functioning, physical role limitations, and general health). Seizures in both non-CNS and CNS tumor survivors increased the risk of unemployment and part-time employment.
Peripheral neuropathy
Vinca alkaloid agents (vincristine and vinblastine) and heavy metals (cisplatin and carboplatin) may cause peripheral neuropathy.[91,92,93]
- Peripheral neuropathy presents during treatment and appears to improve or clinically resolve after completion of therapy.[91] However, recent studies of long-term survivors suggest that chemotherapy-induced peripheral neuropathy is likely under-ascertained.[94]
- Higher cumulative doses of vincristine and/or intrathecal methotrexate have been linked to neuromuscular impairments in long-term survivors of childhood ALL, which suggests that persistent effects of these agents may affect functional status in aging survivors.[91]
- SJLIFE study investigators observed associations between peripheral neuropathy and impairment in performance measures of movement (mobility and walking endurance) and quality of life (physical functioning, role physical, and general health) among survivors of childhood ALL.[95]
- Static-standing balance impairment (a predictor of falling within the next 90 days) was more common in survivors compared with controls but was not associated with peripheral neuropathy.[95]
- Among adult survivors of extracranial solid tumors of childhood (median time from diagnosis, 25 years), standardized assessment of neuromuscular function disclosed motor impairment in association with vincristine exposure and sensory impairment in association with cisplatin exposure.[92]
- Survivors with sensory impairment demonstrated a higher prevalence of functional performance limitations related to poor endurance and mobility restrictions.
Stroke or other cerebrovascular effects
- Childhood CNS tumor survivors have a 43-fold elevated risk of stroke compared with siblings.[42,96]
- Cranial radiation therapy (dose dependent), baseline atherosclerosis, hypertension, and African American ethnicity are identified risk factors.[97,98,99] For information about stroke, see the Cerebrovascular disease section.
- A British CCSS (n = 13,457 survivors) that included 2,885 childhood CNS tumor survivors reported the following:[100]
- CNS tumor survivors who were treated with cranial irradiation had an increased risk of developing cerebrovascular disease between the ages of 50 years and 65 years.
- Compared with an expected incidence of 4.2%, the cumulative incidence of cerebrovascular disease in survivors was 11.6% by age 40 years, 16% by age 50 years, and 26% by age 65 years.
- Among 271 CCSS participants with a history of stroke at a median age of 19 years, 70 reported a second stroke at a median age of 32 years.[101]
- The 10-year cumulative incidence of late recurrent stroke was 21% overall, and 33% for those treated with 50 Gy or higher cranial radiation therapy.
- Risk factors for recurrent stroke included the following:
- Cranial radiation therapy 50 Gy or higher (HR, 4.4; 95% CI, 1.4–13.7).
- Hypertension (HR, 1.9; 95% CI, 1.0–3.5).
- Older age at first stroke (HR, 6.4; 95% CI, 1.8–23).
- A PENTEC review identified 101 of 3,989 pediatric patients who experienced at least one cerebrovascular toxicity (transient ischemic attack, stroke, moyamoya, or arteriopathy).[102]
- Predicted incidences were based on the radiation dose to the Circle of Willis: 0.2% at 30 Gy, 1.3% at 45 Gy, and 4.4% at 54 Gy.
- At an attained age of 35 years, the predicted stroke incidence was 0.9% to 1.3% for 30 Gy, 1.8% to 2.7% for 45 Gy, and 2.8% to 4.1% for 54 Gy (compared with a baseline risk of 0.2%–1.0%).
- At an attained age of 45 years, the predicted stroke incidence was 1.2% to 4.2% for 30 Gy, 4.5% to 8.6% for 45 Gy, and 6.7% to 13% for 54 Gy (compared with a baseline risk of 0.5%–1.0%).
Hypersomnia (daytime sleepiness) or narcolepsy
- In a retrospective review of brain tumor patients treated at SJCRH, investigators identified 39 of 2,336 patients who were diagnosed with hypersomnia/narcolepsy, for a prevalence rate of 1,670 cases per 100,000, which is much higher than a prevalence rate of 20 to 50 cases per 100,000 reported in the general population.[103]
- This may be an underestimate in childhood brain tumor survivors because many patients with mild-to-moderate symptoms, such as fatigue and sleep disturbances, may not be recognized or referred to a sleep specialist.
- Hypersomnia/narcolepsy was diagnosed at a median of 6 years (range, 0.4–13.2 years) from tumor diagnosis and 4.7 years (range, 1.5–10.4 years) from cranial radiation.
- Midline tumor location and antiepilepsy drug use correlated with hypersomnia/narcolepsy, while radiation dose higher than 30 Gy trended toward significance.
- Posterior fossa tumor location was associated with a reduced risk of hypersomnia.
- Treatment of hypersomnia/narcolepsy should be individualized and pharmacologic intervention with stimulants may be beneficial.
- In a baseline evaluation of 82 childhood CNS tumor survivors (median age, 13.8 years) participating in a randomized controlled trial of neurofeedback, 48% of survivors endorsed sleep problems and scored significantly worse than the norm on the Sleep Disturbance Scale for Children in the subscales for initiating and maintaining sleep, excessive somnolence, and total scale.[104]
- Emotional problems and/or hyperactivity/inattention were independent potential risk factors for sleep problems. Sleep problems were also associated with worse parent-reported executive functioning.
Other neurological sequelae
- In a report from the CCSS that compared self-reported neurological late effects among 4,151 adult survivors of childhood ALL with siblings, survivors were at elevated risk for late-onset coordination problems, motor problems, seizures, and headaches.[89]
- The overall cumulative incidence was 44% at 20 years. Serious headaches were most common, with a cumulative incidence of 25.8% at 20 years, followed by focal neurological dysfunction (21.2%) and seizures (7%).
- Children who were treated with regimens that included cranial irradiation for ALL and those who suffered relapse were at increased risk for late-onset neurological sequelae.
- A cross-sectional study evaluated neurological morbidity and quality of life in 162 survivors of childhood ALL (median age at evaluation, 15.7 years; median time from completion of therapy, 7.4 years) in concert with a clinical neurological examination.[105]
- Neurological symptoms were present in 83% of survivors, but symptom-related morbidity was low and quality of life was high in most survivors.
- The most commonly reported symptoms included neuropathy (63%), headache (46.9%), dizziness (33.3%), and back pain (22.8%).
- Female sex, ten doses or more of intrathecal chemotherapy, cranial irradiation, CNS leukemia at diagnosis, and history of ALL relapse were associated with neurological morbidity.
- Neuroimaging studies of irradiated and nonirradiated ALL survivors demonstrate a variety of CNS abnormalities, including leukoencephalopathy, cerebral lacunes, cerebral atrophy, and dystrophic calcifications (mineralizing microangiopathy).[52,58,106,107]
- Among these, abnormalities of cerebral white matter integrity and volume have been correlated with neurocognitive outcomes.
- Cavernomas have also been observed in ALL survivors treated with cranial irradiation. They have been speculated to result from angiogenic processes as opposed to tumorigenesis.[108]
- In a retrospective cross-sectional study, 101 survivors of childhood ALL (mean time since diagnosis, 27.6 years) who were treated with cranial irradiation (63.4% received ≤18 Gy) underwent neurocognitive testing and 3T brain MRI.[109]
- Small focal intracerebral hemorrhages that are only visible on exquisitely sensitive MRI sequences were identified and localized using susceptibility weighted imaging.
- At least one microbleed was present in 85% of survivors, and they occurred more frequently in frontal lobes.
- A radiation dose of 24 Gy conveyed a fivefold greater risk of having multiple microbleeds when compared with a dose of 18 Gy.
- No significant difference was found in neurocognitive scores with either the absence or presence of microbleeds or their location.
- CCSS investigators evaluated treatment-related neurological sequelae in survivors of childhood CNS tumors.[88]
- In 1,876 5-year survivors of CNS tumors from the CCSS, the cumulative incidence of headaches increased from 38% at 5 years to 53% at 30 years since diagnosis.
- Coordination problems increased from 21% at 5 years to 53% at 30 years since diagnosis, and motor impairment increased from 21% to 35% during this same time period.
- Increased risk of motor impairment was associated with tumor recurrence (HR, 2.6), development of a meningioma (HR, 2.3), and stroke (HR, 14.9).
- The cumulative incidence of hearing loss increased from 9% at 5 years to 23% at 30 years, cumulative incidence of tinnitus increased from 8% at 5 years to 21% at 30 years, and cumulative incidence of vertigo increased from 9% at 5 years to 17% at 30 years.
- Risks of motor impairment (HR, 7.6) and hearing loss (HR, 18.4) were elevated compared with siblings.
- CCSS investigators estimated the prevalence and cumulative incidence of neuromuscular dysfunction (motor or sensory dysfunction) among 25,583 childhood cancer survivors and 5,044 siblings.[110]
- Neuromuscular dysfunction was prevalent in 14.7% of survivors 5 years after diagnosis, compared with 1.5% in siblings (prevalence ratio [PR], 9.9%; 95% CI, 7.9–12.4).
- The prevalence of neuromuscular dysfunction was highest in survivors of CNS tumors (PR, 27.6; 95% CI, 22.1–34.6) and sarcomas (PR, 11.5; 95% CI, 9.1–14.5).
- The 20-year cumulative incidence increased to 24.3% in survivors 20 years after diagnosis.
- Cancer treatments associated with increased prevalence of neuromuscular dysfunction included spinal radiation therapy, increasing doses of cranial radiation therapy, and platinum agents.
- Neuromuscular dysfunction was associated with downstream adverse outcomes, including concurrent or later obesity (PR, 1.1; 95% CI, 1.1–1.2), anxiety (PR, 2.5; 95% CI, 2.2–2.9), depression (PR, 2.1; 95% CI, 1.9–2.3), and lower likelihood of graduating college (PR, 0.92; 95% CI, 0.90–0.94) and finding employment (PR, 0.8; 95% CI, 0.8–0.9).
Table 4 summarizes CNS late effects and the related health screenings.
Table 4. Central Nervous System Late Effectsa
Predisposing Therapy |
Neurological Effects |
Health Screening |
IQ = intelligence quotient; IT = intrathecal; IV = intravenous. |
a Adapted from theChildren's Oncology Group Long-Term Follow-Up Guidelines for Survivors of Childhood, Adolescent, and Young Adult Cancers. |
Heavy metals (carboplatin, cisplatin) |
Peripheral sensory neuropathy |
Neurological examination |
Vinca alkaloid agents (vinblastine, vincristine) |
Peripheral sensory or motor neuropathy (areflexia, weakness, foot drop, paresthesias) |
Neurological examination |
Methotrexate (high dose IV or IT); cytarabine (high dose IV or IT); radiation exposing the brain |
Clinical leukoencephalopathy (spasticity, ataxia, dysarthria, dysphagia, hemiparesis, seizures); headaches; seizures; sensory deficits |
History: cognitive, motor, and/or sensory deficits, seizures |
Neurological examination |
Radiation exposing cerebrovascular structures |
Cerebrovascular complications (stroke, Moyamoya disease, occlusive cerebral vasculopathy) |
History: transient/permanent neurological events |
Blood pressure test |
Neurological examination |
Neurosurgery–brain |
Motor and/or sensory deficits (paralysis, movement disorders, ataxia, eye problems [ocular nerve palsy, gaze paresis, nystagmus, papilledema, optic atrophy]); seizures |
Neurological examination |
Neurology evaluation |
Neurosurgery–brain |
Hydrocephalus; shunt malfunction |
Abdominal x-ray |
Neurosurgery evaluation |
Neurosurgery–spine |
Neurogenic bladder; urinary incontinence |
History: hematuria, urinary urgency/frequency, urinary incontinence/retention, dysuria, nocturia, abnormal urinary stream |
Neurosurgery–spine |
Neurogenic bowel; fecal incontinence |
History: chronic constipation, fecal soiling |
Rectal examination |
Predisposing Therapy
|
Neurocognitive Effects
|
Health Screening
|
Methotrexate (high-dose IV or IT); cytarabine (high-dose IV or IT); radiation exposing the brain; neurosurgery–brain |
Neurocognitive deficits (executive function, memory, attention, processing speed, etc.); learning deficits; diminished IQ; behavioral change |
Assessment of educational and vocational progress |
Formal neuropsychological evaluation |
Psychosocial
Many childhood cancer survivors report reduced quality of life, impaired health status, or other adverse psychosocial outcomes, compared with siblings or noncancer population groups.[111,112] Vulnerable groups have been identified related to sociodemographic factors (e.g., female sex), specific cancer diagnoses (e.g., CNS tumor), cancer treatments (e.g., cranial radiation therapy), health behaviors (e.g., smoking), and type/cumulative burden of chronic health conditions. The diagnosis of childhood cancer may also affect psychosocial outcomes and the expected attainment of functional and social independence in adulthood. Several investigations have demonstrated that survivors of pediatric CNS tumors are particularly vulnerable.[48,113]
Evidence for adverse psychosocial adjustment after childhood cancer has been derived from sources, ranging from patient-reported or proxy-reported outcomes to data from population-based registries. The former may be limited by small sample size, cohort selection and participation bias, and variable methods and venues (clinical vs. distance-based survey) of assessments. The latter is often not well correlated with clinical and treatment characteristics that permit the identification of survivors at high risk of psychosocial deficits.
Achievement of social milestones
Survivors with neurocognitive deficits are particularly vulnerable to deficits in achievement of expected social outcomes during adulthood.
- In a population-based study of adult survivors of CNS tumors diagnosed in childhood or adolescence, survivors had significantly poorer self-perception and self-esteem than did individuals in the general population. Female sex, persistent visible physical sequelae, specific tumor type, and treatment with cranial radiation therapy predicted poor self-perception outcomes.[114]
- In a series of CNS malignancy survivors (n = 802) reported from the CCSS, adverse outcome on multiple indicators of successful adult adjustment (educational achievement, income, employment, and marital status) were most prevalent among survivors who reported neurocognitive dysfunction.[41]
- Collectively, studies evaluating psychosocial outcomes among CNS tumor survivors indicate deficits in social competence that worsen over time.[115] This includes problems with peer rejection and isolation in childhood/adolescence, and the inability to develop friendships and romantic relationships as adults.
- In a CCSS study that evaluated predictors of independent living status across diagnostic groups, adult survivors of childhood cancer with neurocognitive, psychological, or physical late effects were less likely to live independently as adults than were siblings in the comparison group.[116]
- In an SJLIFE study of 224 survivors of CNS tumors (median current age, 26 years; median time from diagnosis, 18 years), neurocognitive impairment was significantly associated with lower educational attainment, unemployment, and dependent living.[3]
- Among one subgroup, significant impairments in social perceptions skills, including affect recognition, contributed to limited achievement of functional outcomes. Self-reports of social adjustment were generally within normal limits, suggesting limited self-insight into social deficits.[117]
- In a series of 1,560 adolescent survivors of childhood ALL treated with chemotherapy alone, the CCSS identified a significant proportion of survivors who still experienced problems with headstrong behavior, inattention-hyperactivity, and social withdrawal, which were associated with an increased risk of special education placement and predicted reduced adult educational attainment.[69]
- In a cross-sectional study of 855 childhood leukemia survivors (mean, 10.2 years from diagnosis) in the Leucémie de l'Enfant et de l'Adolescent (LEA) cohort, investigators identified independent factors associated with repeating a grade in school, including low parental education and household financial difficulties, emphasizing the importance of considering socioeconomic factors that may affect access to educational support. Survivors who were adolescents (aged 11–17 years) at diagnosis were also at greater risk than were survivors who were children (aged <11 years) at diagnosis.[118]
- In a population-based, cross-sectional, survivorship study, 28% of adolescent and young adult (AYA) survivors of sarcoma (aged 18–39 years at diagnosis; median time from diagnosis, 6.2 years) experienced impaired social functioning.[119] Multivariable analysis identified independent associations with impaired social functioning and unemployment (OR, 3.72; 95% CI, 1.26–10.97) and having to make lifestyle changes because of financial problems caused by an individual's physical condition or medical treatment (OR, 3.39; 95% CI, 1.12–10.30). In contrast, better social support reduced the risk of impaired social functioning (OR, 0.74; 95% CI, 0.57–0.96).
Psychological distress and suicidality
Childhood cancer survivors are also at risk of developing symptoms of psychological distress and suicidality.[120]
- The SJLIFE study was used to compare the risks of suicidal ideation, suicidal behaviors, and mortality in adult survivors of childhood cancer with those of the general population.[121]
- Survivors (n = 3,096) reported a similar 12-month prevalence of suicidal ideation compared with the general population (standardized incidence ratio [SIR], 0.68) and a lower prevalence of suicidal behaviors (planning: SIR, 0.17; attempts: SIR, 0.07) and mortality (standardized mortality ratio, 0.60).
- Among survivors, depression (RR, 12.30), anxiety (RR, 2.19), and financial stress (RR, 1.47) were found to be risk factors associated with suicidal ideation.
- Survivors who were currently single, widowed, or divorced; who were not working full time; who reported financial stress during the previous year; and who reported sleep disturbances had an approximately 30% to 50% higher prevalence of suicidal ideation.
- A CCSS study evaluated the prevalence of recurrent suicidal ideation among 9,128 adult long-term survivors of childhood cancer.[122]
- Survivors were more likely to report late suicidal ideation (OR, 1.9; 95% CI, 1.5–2.5) and recurrent suicidal ideation (OR, 2.6; 95% CI, 1.8–3.8) compared with siblings.
- History of seizure was associated with a twofold increased likelihood of suicide ideation in survivors.
- A population-based study evaluated suicide among adults treated for cancer before age 25 years.[123]
- The absolute risk of suicide was low (24 cases among 3,375 deaths).
- The HR of suicide was increased among individuals treated for cancer in childhood (0–14 years; HR, 2.5; 95% CI, 1.7–3.8) and in adolescence and young adulthood (15–24 years; HR, 2.3; 95% CI, 1.2–4.6).
The presence of chronic health conditions can also impact aspects of psychological health.
- In a study that evaluated psychological outcomes among long-term survivors treated with HSCT, 22% of survivors and 8% of sibling controls reported adverse outcomes. Somatic distress was the most prevalent condition and affected 15% of HSCT survivors, representing a threefold higher risk compared with siblings. HSCT survivors with severe or life-threatening health conditions and active chronic GVHD had a twofold increased risk of somatic distress.[124]
- A report from the CCSS revealed that the presence of chronic pulmonary, endocrine, and cardiac conditions was associated with increased risk of psychological distress symptoms in a sample of 5,021 adult survivors of childhood cancer.[125]
- In a CCSS investigation that evaluated long-term psychological and educational outcomes among survivors of neuroblastoma, survivors demonstrated elevated risks of psychological impairment, which was associated with the use of special education services and lower educational attainment. The presence of two or more chronic health conditions, but not common treatment exposures, predicted psychological impairment. Specifically, pulmonary disease predicted impairment in all five psychological domains, whereas endocrine disease and peripheral neuropathy each predicted impairment in three domains.[126]
Incorporation of psychological screening into clinical visits for childhood cancer survivors may be valuable. However, limiting such evaluations to those returning to long-term follow-up clinics may result in a biased subsample of survivors with more difficulties, and precise prevalence rates may be difficult to establish.
- In a study of 101 adult survivors of childhood cancer, psychological screening was performed during a routine annual evaluation at the survivorship clinic at the Dana Farber Cancer Institute.[127]
- On the Symptom Checklist 90 Revised, 32 survivors had a positive screen (indicating psychological distress), and 14 survivors reported at least one suicidal symptom.
- Risk factors for psychological distress included survivors' dissatisfaction with physical appearance, poor physical health, and treatment with cranial irradiation.
- This instrument was shown to be feasible for use in the clinic-visit setting because the psychological screening was completed in less than 30 minutes and did not appear to cause distress in the survivors in 80% of cases.
For more information about psychological distress, depression, and cancer patients, see Adjustment to Cancer: Anxiety and Distress and Depression.
Serious mental illnesses
A population-based study from Taiwan compared the prevalence of serious mental illnesses in 5,121 childhood and adolescent cancer survivors with that of population controls.[128]
- Survivors (mean age, 9 years; 60% were 5 or more years from diagnosis) showed increased risks of six serious mental illnesses compared with controls. These disorders included the following:
- Autism spectrum disorder (HR, 10.42; 95% CI, 4.58–23.69).
- ADHD (HR, 6.59; 95% CI, 4.91–8.86).
- Bipolar disorder (HR, 2.93; 95% CI, 1.26–6.80).
- Major depressive disorder (HR, 1.88; 95% CI, 1.26–2.79).
- Obsessive-compulsive disorder (OCD) (HR, 3.37; 95% CI, 1.33–8.52).
- Post-traumatic stress disorder (PTSD) (HR, 6.10; 95% CI, 1.46–25.54).
- Survivors were younger than controls at the time of diagnosis of ADHD, schizophrenia, major depressive disorder, and OCD.
- Risks of serious mental illnesses varied according to specific cancer types. The greatest number of major psychiatric disorders was observed among survivors of brain cancer and lymphatic/hematopoietic tissue cancer.
A population-based study linked individuals with a history of six common cancers diagnosed at age 15 to 21 years to provincial health care data to compare rates of outpatient (family physician and psychiatrist) visits for psychiatric indications and time to severe psychiatric events (emergency room visit, hospitalization, and suicide). The study included 2,208 AYA cancer patients and 10,457 matched controls.[129]
- Five-year AYA survivors experienced higher rates of outpatient mental health visits than did controls (671 vs. 506 visits per 1,000 person-years; RR, 1.3), higher risk of a severe psychiatric episode (HR, 1.2), and higher risk of a psychotic disorder–associated severe event (HR, 2.0).
- Treatment in an adult center was associated with substantially higher outpatient visit rates compared with treatment in pediatric settings (RR, 1.8).
PTSD after childhood cancer
Despite the many stresses associated with the diagnosis of cancer and its treatment, studies have generally shown low levels of post-traumatic stress symptoms and PTSD in children with cancer, typically no higher than those in healthy comparison children.[130]
- The prevalence of PTSD and post-traumatic stress symptoms has been reported in 15% to 20% of young adult survivors of childhood cancer, with estimates varying based on criteria used to define these conditions.[131]
- In a cohort of 5,121 childhood and adolescent cancer survivors (mean age, 9 years), the incidence of PTSD was 0.59%. Compared with controls, survivors showed a 6.10-fold elevated risk of being diagnosed with PTSD. Brain cancer survivors had the highest risk of all cancer diagnoses for PTSD (HR, 18.50; 95% CI, 2.11–162.64).[128]
- Patient and parent adaptive style appear to be significant determinants of PTSD in the pediatric oncology setting.[132,133]
- Survivors with PTSD reported more psychological problems and negative beliefs about their illness and health status than did those without PTSD.[134,135]
- A subset of adult survivors (9%) from the CCSS reported functional impairment and/or clinical distress in addition to the set of symptoms consistent with a full diagnosis of PTSD.[136]
- PTSD was significantly more prevalent in survivors than in sibling comparisons.
- PTSD was significantly associated with being unmarried, having an annual income of less than $20,000, being unemployed, having a high school education or less, and being older than 30 years.
- Survivors who were treated with cranial irradiation before age 4 years were at particularly higher risk of developing PTSD.
- Intensive cancer-directed therapy was also associated with increased risk of full PTSD.
- Because avoidance of places and persons associated with the cancer is part of PTSD, the syndrome may interfere with obtaining appropriate health care.[137]
- Those with PTSD perceive greater current threats to their lives or the lives of their children.
- Other risk factors for PTSD include poor family functioning, decreased social support, and noncancer stressors.
Psychosocial outcomes among childhood, adolescent, and young adult cancer survivors
Most research on late effects after cancer has focused on individuals with a cancer diagnosis during childhood. Little is known about the specific impact of a cancer diagnosis with an onset in adolescence or the impact of childhood cancer on AYA psychosocial outcomes.
Evidence (psychosocial outcomes in AYA cancer survivors):
- Adult survivors of cancer diagnosed during adolescence (aged 15–18 years) (N = 825) were compared with an age-matched sample from the general population and a comparison group of adults without cancer.[138]
- Female survivors of adolescent cancers achieved fewer developmental milestones related to their psychosexual development, such as having their first boyfriend, or they reached these milestones later.
- Male survivors were more likely to live with their parents than were same-sex controls.
- Adolescent cancer survivors were less likely to have ever married or have had children. Survivors were significantly older at their first marriage and at the birth of their first child than were their age-matched samples.
- Survivors in this cohort were also significantly less satisfied with their general and health-related life than were people in a community-based control group. Impaired general and health-related life satisfaction were associated with somatic late effects, symptoms of depression and anxiety, and lower rates of posttraumatic growth.[139]
- A survey of 4,054 AYA cancer survivors and 345,592 respondents who had no history of cancer reported the following:[140]
- AYA cancer survivors were more likely to smoke (26% vs. 18%), be affected by obesity (31% vs. 27%), and have chronic conditions such as cardiovascular disease (14% vs. 7%), hypertension (35% vs. 9%), asthma (15% vs. 8%), disability (36% vs. 18%), and poor mental health (20% vs. 10%).
- They were also less likely to receive medical care because of cost (24% vs. 15%).
- The CCSS evaluated outcomes of 2,979 adolescent survivors and 649 siblings of childhood cancer survivors to determine the incidence of difficulty in six behavioral and social domains (depression/anxiety, being headstrong, attention deficit, peer conflict/social withdrawal, antisocial behaviors, and social competence).[141]
- Survivors were 1.5 times (95% CI, 1.1–2.1) more likely than siblings to have symptoms of depression/anxiety and 1.7 times (95% CI, 1.3–2.2) more likely than siblings to have antisocial behaviors.
- Scores in the depression/anxiety, attention deficit, and antisocial domains were significantly elevated in adolescents treated for leukemia or CNS tumors, compared with the scores in siblings.
- In addition, survivors of neuroblastoma had difficulty in the depression/anxiety and antisocial domains.
- CNS-directed treatments (cranial radiation therapy and/or intrathecal methotrexate) were specific risk factors for adverse behavioral outcomes.
- Another CCSS study evaluated psychological and neurocognitive function in 2,589 long-term cancer survivors who were diagnosed during adolescence and young adulthood.[142]
- Compared with a sibling cohort, survivors diagnosed during adolescence and young adulthood reported higher rates of depression (OR, 1.55; 95% CI, 1.04–2.30) and anxiety (OR, 2.00; 95% CI, 1.17–3.43) and reported more cognitive problems affecting task efficiency (OR, 1.72; 95% CI, 1.21–2.43), emotional regulation (OR, 1.74; 95% CI, 1.26–2.40), and memory (OR, 1.44; 95% CI, 1.09–1.89).
- Survivors of lymphoma and sarcoma diagnosed during later adolescence were at reduced risk of psychosocial and neurocognitive problems than were those diagnosed before age 11 years. These outcomes did not differ by age at diagnosis among CNS tumor and leukemia survivors.
- Survivors diagnosed during adolescence and young adulthood were also significantly less likely than sibling controls to have attained a post–high school education, be working full time, be married, or be living independently; inferior social outcomes were related to neurocognitive symptoms.
- A follow-up CCSS study evaluated profiles of symptom comorbidities in 3,993 adolescents (aged 13–17 years) treated for cancer.[143] Latent profile analysis identified four symptom profiles:
- No significant symptoms.
- Elevated internalizing symptoms (anxiety and/or depression, social withdrawal, and attention problems).
- Elevated externalizing symptoms (headstrong behavior and attention problems).
- Elevated internalizing and externalizing symptoms.
Overall results support that behavioral, emotional, and social symptoms frequently co-occur and are associated with treatment exposures (cranial radiation, corticosteroids, and methotrexate) and late effects (obesity, cancer-related pain, and sensory impairments) in adolescent survivors diagnosed between 1970 and 1986.
- Another CCSS study characterized the prevalence and risk of pain, clinically significant interference in daily activities because of pain, and recurrent pain in 10,012 adult survivors of childhood cancer (median time since diagnosis, 23 years).[144]
- A significant minority of survivors endorsed moderate to severe pain (29%), moderate to extreme pain interference (20%), and moderate to severe recurrent pain (9%).
- Older age at diagnosis and follow-up, female sex, and presence of grades 3 to 4 chronic medical conditions were consistently associated with an increased risk of worse pain outcomes.
- Minority racial and ethnic groups, diagnosis of CNS tumor, and treatment with platinum-based chemotherapy and cranial radiation were associated with an increased risk of late-occurrence pain and pain interference.
- Depression and anxiety were associated with increased risk of all pain outcomes, and poor vitality mediated the effects of anxiety on high pain and pain interference.
- An SJLIFE study evaluated the associations between comprehensive medical, neurocognitive, and physical performance assessments and self-reported pain, quality of life, and social functioning in 2,836 survivors (mean age, 32.2 years; mean time since diagnosis, 23.7 years) and 343 noncancer community controls.[145]
- Moderate-to-very-severe pain with moderate-to-extreme daily interference was endorsed by 18% of survivors, compared with 8% of controls (P < .001).
- Survivors of soft tissue sarcoma (OR, 9.25), non-Hodgkin lymphoma (OR, 4.13), and Ewing sarcoma and/or osteosarcoma (OR, 3.93) had the highest odds of pain with daily interference, compared with controls.
- In multivariable models that included treatment exposures, histories of amputation (OR, 1.89) and/or limb-sparing surgery (OR, 2.30) were associated with increased odds of pain with daily interference.
- Pain with daily interference was associated with an increased risk of impaired neurocognition (attention: RR, 1.88; memory: RR, 1.65) and physical functioning (aerobic capacity: RR, 2.29; mobility: RR, 1.71).
- Pain with daily interference was also associated with an increased risk of impaired social functioning (inability to hold a job and/or attend school: RR, 4.46; assistance with routine and/or personal care needs: RR, 5.64), and health-related quality of life (physical: RR, 6.34; emotional: RR, 2.83).
- A Nordic, register-based cohort study evaluated whether childhood cancer survivors (n = 18,621) were at a higher risk of developing psychiatric disorders later in life than their siblings (n = 24,775) and the general population (n = 88,630).[146]
- The cumulative incidence of contact with a psychiatric hospital by age 30 years was 15.9% for childhood cancer survivors, 14.0% for siblings, and 12.7% for matched (by birth year, sex, and country/municipality) population controls.
- The absolute difference was small, but survivors were still at a higher RR for any psychiatric hospital contact than their siblings (1.39) and matched controls (HR, 1.34).
Evidence (functional and social independence):
- In a study of 665 survivors of CNS tumors (54% male; 52% treated with cranial radiation therapy; median age, 15 years; and 12 years from diagnosis), CCSS investigators observed the following:[113]
- Almost 50% of survivors experienced social difficulties related to peer relationships that exceeded those of survivors of solid tumors and sibling controls.
- Cranial radiation exposure predicted impaired social and peer relationships, and cognitive impairment mediated these associations.
- An SJLIFE study investigated functional and social independence in 306 CNS tumor survivors (astrocytoma [n = 130], medulloblastoma [n = 77], ependymoma [n = 36], and other [n = 63]; median age, 25 years; and time since diagnosis, 16.8 years).[48]
- Only 40% of long-term survivors in the study cohort achieved complete independence as adults.
- Predictors of nonindependence included treatment with craniospinal irradiation, history of hydrocephalus with shunting, and younger age at diagnosis.
- Beyond impaired IQ scores, functional limitations in aerobic capacity, flexibility, and adaptive physical function were significantly associated with nonindependence.
References:
-
Grill J, Kieffer V, Kalifa C: Measuring the neuro-cognitive side-effects of irradiation in children with brain tumors. Pediatr Blood Cancer 42 (5): 452-6, 2004.
-
Marusak HA, Iadipaolo AS, Harper FW, et al.: Neurodevelopmental consequences of pediatric cancer and its treatment: applying an early adversity framework to understanding cognitive, behavioral, and emotional outcomes. Neuropsychol Rev 28 (2): 123-175, 2018.
-
Brinkman TM, Krasin MJ, Liu W, et al.: Long-Term Neurocognitive Functioning and Social Attainment in Adult Survivors of Pediatric CNS Tumors: Results From the St Jude Lifetime Cohort Study. J Clin Oncol 34 (12): 1358-67, 2016.
-
Moxon-Emre I, Bouffet E, Taylor MD, et al.: Impact of craniospinal dose, boost volume, and neurologic complications on intellectual outcome in patients with medulloblastoma. J Clin Oncol 32 (17): 1760-8, 2014.
-
Krull KR, Zhang N, Santucci A, et al.: Long-term decline in intelligence among adult survivors of childhood acute lymphoblastic leukemia treated with cranial radiation. Blood 122 (4): 550-3, 2013.
-
Annett RD, Hile S, Bedrick E, et al.: Neuropsychological functioning of children treated for acute lymphoblastic leukemia: impact of whole brain radiation therapy. Psychooncology 24 (2): 181-9, 2015.
-
van der Plas E, Qiu W, Nieman BJ, et al.: Sex-Specific Associations Between Chemotherapy, Chronic Conditions, and Neurocognitive Impairment in Acute Lymphoblastic Leukemia Survivors: A Report From the Childhood Cancer Survivor Study. J Natl Cancer Inst 113 (5): 588-596, 2021.
-
Krull KR, Khan RB, Ness KK, et al.: Symptoms of attention-deficit/hyperactivity disorder in long-term survivors of childhood leukemia. Pediatr Blood Cancer 57 (7): 1191-6, 2011.
-
Kahalley LS, Conklin HM, Tyc VL, et al.: ADHD and secondary ADHD criteria fail to identify many at-risk survivors of pediatric ALL and brain tumor. Pediatr Blood Cancer 57 (1): 110-8, 2011.
-
Mahajan A, Stavinoha PL, Rongthong W, et al.: Neurocognitive Effects and Necrosis in Childhood Cancer Survivors Treated With Radiation Therapy: A PENTEC Comprehensive Review. Int J Radiat Oncol Biol Phys 119 (2): 401-416, 2024.
-
Cheung YT, Brinkman TM, Li C, et al.: Chronic Health Conditions and Neurocognitive Function in Aging Survivors of Childhood Cancer: A Report from the Childhood Cancer Survivor Study. J Natl Cancer Inst 110 (4): 411-419, 2018.
-
Bass JK, Liu W, Banerjee P, et al.: Association of Hearing Impairment With Neurocognition in Survivors of Childhood Cancer. JAMA Oncol 6 (9): 1363-1371, 2020.
-
Barlow-Krelina E, Chen Y, Yasui Y, et al.: Consistent Physical Activity and Future Neurocognitive Problems in Adult Survivors of Childhood Cancers: A Report From the Childhood Cancer Survivor Study. J Clin Oncol 38 (18): 2041-2052, 2020.
-
Bernal JDK, Recchia F, Yu DJ, et al.: Physical activity and exercise for cancer-related cognitive impairment among individuals affected by childhood cancer: a systematic review and meta-analysis. Lancet Child Adolesc Health 7 (1): 47-58, 2023.
-
Phillips NS, Stratton KL, Williams AM, et al.: Late-onset Cognitive Impairment and Modifiable Risk Factors in Adult Childhood Cancer Survivors. JAMA Netw Open 6 (5): e2316077, 2023.
-
Merchant TE, Schreiber JE, Wu S, et al.: Critical combinations of radiation dose and volume predict intelligence quotient and academic achievement scores after craniospinal irradiation in children with medulloblastoma. Int J Radiat Oncol Biol Phys 90 (3): 554-61, 2014.
-
Armstrong GT, Conklin HM, Huang S, et al.: Survival and long-term health and cognitive outcomes after low-grade glioma. Neuro Oncol 13 (2): 223-34, 2011.
-
Di Pinto M, Conklin HM, Li C, et al.: Learning and memory following conformal radiation therapy for pediatric craniopharyngioma and low-grade glioma. Int J Radiat Oncol Biol Phys 84 (3): e363-9, 2012.
-
Ris MD, Walsh K, Wallace D, et al.: Intellectual and academic outcome following two chemotherapy regimens and radiotherapy for average-risk medulloblastoma: COG A9961. Pediatr Blood Cancer 60 (8): 1350-7, 2013.
-
Bowers DC, Liu Y, Leisenring W, et al.: Late-occurring stroke among long-term survivors of childhood leukemia and brain tumors: a report from the Childhood Cancer Survivor Study. J Clin Oncol 24 (33): 5277-82, 2006.
-
Nassar SL, Conklin HM, Zhou Y, et al.: Neurocognitive outcomes among children who experienced seizures during treatment for acute lymphoblastic leukemia. Pediatr Blood Cancer 64 (8): , 2017.
-
Torres VA, Ashford JM, Wright E, et al.: The impact of socioeconomic status (SES) on cognitive outcomes following radiotherapy for pediatric brain tumors: a prospective, longitudinal trial. Neuro Oncol 23 (7): 1173-1182, 2021.
-
Mabbott DJ, Spiegler BJ, Greenberg ML, et al.: Serial evaluation of academic and behavioral outcome after treatment with cranial radiation in childhood. J Clin Oncol 23 (10): 2256-63, 2005.
-
Brière ME, Scott JG, McNall-Knapp RY, et al.: Cognitive outcome in pediatric brain tumor survivors: delayed attention deficit at long-term follow-up. Pediatr Blood Cancer 50 (2): 337-40, 2008.
-
Edelstein K, Spiegler BJ, Fung S, et al.: Early aging in adult survivors of childhood medulloblastoma: long-term neurocognitive, functional, and physical outcomes. Neuro Oncol 13 (5): 536-45, 2011.
-
Palmer SL, Goloubeva O, Reddick WE, et al.: Patterns of intellectual development among survivors of pediatric medulloblastoma: a longitudinal analysis. J Clin Oncol 19 (8): 2302-8, 2001.
-
Mulhern RK, Merchant TE, Gajjar A, et al.: Late neurocognitive sequelae in survivors of brain tumours in childhood. Lancet Oncol 5 (7): 399-408, 2004.
-
Brinkman TM, Reddick WE, Luxton J, et al.: Cerebral white matter integrity and executive function in adult survivors of childhood medulloblastoma. Neuro Oncol 14 (Suppl 4): iv25-36, 2012.
-
Jacola LM, Ashford JM, Reddick WE, et al.: The relationship between working memory and cerebral white matter volume in survivors of childhood brain tumors treated with conformal radiation therapy. J Neurooncol 119 (1): 197-205, 2014.
-
Palmer SL, Glass JO, Li Y, et al.: White matter integrity is associated with cognitive processing in patients treated for a posterior fossa brain tumor. Neuro Oncol 14 (9): 1185-93, 2012.
-
Ali JS, Ashford JM, Swain MA, et al.: Predictors of Cognitive Performance Among Infants Treated for Brain Tumors: Findings From a Multisite, Prospective, Longitudinal Trial. J Clin Oncol 39 (21): 2350-2358, 2021.
-
Merchant TE, Conklin HM, Wu S, et al.: Late effects of conformal radiation therapy for pediatric patients with low-grade glioma: prospective evaluation of cognitive, endocrine, and hearing deficits. J Clin Oncol 27 (22): 3691-7, 2009.
-
Liu APY, Hastings C, Wu S, et al.: Treatment burden and long-term health deficits of patients with low-grade gliomas or glioneuronal tumors diagnosed during the first year of life. Cancer 125 (7): 1163-1175, 2019.
-
Palmer SL, Armstrong C, Onar-Thomas A, et al.: Processing speed, attention, and working memory after treatment for medulloblastoma: an international, prospective, and longitudinal study. J Clin Oncol 31 (28): 3494-500, 2013.
-
Knight SJ, Conklin HM, Palmer SL, et al.: Working memory abilities among children treated for medulloblastoma: parent report and child performance. J Pediatr Psychol 39 (5): 501-11, 2014.
-
Schreiber JE, Gurney JG, Palmer SL, et al.: Examination of risk factors for intellectual and academic outcomes following treatment for pediatric medulloblastoma. Neuro Oncol 16 (8): 1129-36, 2014.
-
Khan RB, Patay Z, Klimo P, et al.: Clinical features, neurologic recovery, and risk factors of postoperative posterior fossa syndrome and delayed recovery: a prospective study. Neuro Oncol 23 (9): 1586-1596, 2021.
-
Schreiber JE, Palmer SL, Conklin HM, et al.: Posterior fossa syndrome and long-term neuropsychological outcomes among children treated for medulloblastoma on a multi-institutional, prospective study. Neuro Oncol 19 (12): 1673-1682, 2017.
-
Moxon-Emre I, Taylor MD, Bouffet E, et al.: Intellectual Outcome in Molecular Subgroups of Medulloblastoma. J Clin Oncol 34 (34): 4161-4170, 2016.
-
Acharya S, Wu S, Ashford JM, et al.: Association between hippocampal dose and memory in survivors of childhood or adolescent low-grade glioma: a 10-year neurocognitive longitudinal study. Neuro Oncol 21 (9): 1175-1183, 2019.
-
Ellenberg L, Liu Q, Gioia G, et al.: Neurocognitive status in long-term survivors of childhood CNS malignancies: a report from the Childhood Cancer Survivor Study. Neuropsychology 23 (6): 705-17, 2009.
-
Armstrong GT, Liu Q, Yasui Y, et al.: Long-term outcomes among adult survivors of childhood central nervous system malignancies in the Childhood Cancer Survivor Study. J Natl Cancer Inst 101 (13): 946-58, 2009.
-
Ris MD, Leisenring WM, Goodman P, et al.: Neuropsychological and socioeconomic outcomes in adult survivors of pediatric low-grade glioma. Cancer 125 (17): 3050-3058, 2019.
-
Shabason EK, Brodsky C, Baran J, et al.: Clinical diagnosis of attention-deficit/hyperactivity disorder in survivors of pediatric brain tumors. J Neurooncol 143 (2): 305-312, 2019.
-
Brinkman TM, Palmer SL, Chen S, et al.: Parent-reported social outcomes after treatment for pediatric embryonal tumors: a prospective longitudinal study. J Clin Oncol 30 (33): 4134-40, 2012.
-
Moyer KH, Willard VW, Gross AM, et al.: The impact of attention on social functioning in survivors of pediatric acute lymphoblastic leukemia and brain tumors. Pediatr Blood Cancer 59 (7): 1290-5, 2012.
-
Mitby PA, Robison LL, Whitton JA, et al.: Utilization of special education services and educational attainment among long-term survivors of childhood cancer: a report from the Childhood Cancer Survivor Study. Cancer 97 (4): 1115-26, 2003.
-
Brinkman TM, Ness KK, Li Z, et al.: Attainment of Functional and Social Independence in Adult Survivors of Pediatric CNS Tumors: A Report From the St Jude Lifetime Cohort Study. J Clin Oncol 36 (27): 2762-2769, 2018.
-
Kahalley LS, Ris MD, Grosshans DR, et al.: Comparing Intelligence Quotient Change After Treatment With Proton Versus Photon Radiation Therapy for Pediatric Brain Tumors. J Clin Oncol 34 (10): 1043-9, 2016.
-
Pulsifer MB, Sethi RV, Kuhlthau KA, et al.: Early Cognitive Outcomes Following Proton Radiation in Pediatric Patients With Brain and Central Nervous System Tumors. Int J Radiat Oncol Biol Phys 93 (2): 400-7, 2015.
-
Kahalley LS, Peterson R, Ris MD, et al.: Superior Intellectual Outcomes After Proton Radiotherapy Compared With Photon Radiotherapy for Pediatric Medulloblastoma. J Clin Oncol 38 (5): 454-461, 2020.
-
Reddick WE, Glass JO, Helton KJ, et al.: Prevalence of leukoencephalopathy in children treated for acute lymphoblastic leukemia with high-dose methotrexate. AJNR Am J Neuroradiol 26 (5): 1263-9, 2005.
-
Waber DP, Queally JT, Catania L, et al.: Neuropsychological outcomes of standard risk and high risk patients treated for acute lymphoblastic leukemia on Dana-Farber ALL consortium protocol 95-01 at 5 years post-diagnosis. Pediatr Blood Cancer 58 (5): 758-65, 2012.
-
Krull KR, Brinkman TM, Li C, et al.: Neurocognitive outcomes decades after treatment for childhood acute lymphoblastic leukemia: a report from the St Jude lifetime cohort study. J Clin Oncol 31 (35): 4407-15, 2013.
-
Reddick WE, Shan ZY, Glass JO, et al.: Smaller white-matter volumes are associated with larger deficits in attention and learning among long-term survivors of acute lymphoblastic leukemia. Cancer 106 (4): 941-9, 2006.
-
Kadan-Lottick NS, Zeltzer LK, Liu Q, et al.: Neurocognitive functioning in adult survivors of childhood non-central nervous system cancers. J Natl Cancer Inst 102 (12): 881-93, 2010.
-
Schuitema I, Deprez S, Van Hecke W, et al.: Accelerated aging, decreased white matter integrity, and associated neuropsychological dysfunction 25 years after pediatric lymphoid malignancies. J Clin Oncol 31 (27): 3378-88, 2013.
-
Armstrong GT, Reddick WE, Petersen RC, et al.: Evaluation of memory impairment in aging adult survivors of childhood acute lymphoblastic leukemia treated with cranial radiotherapy. J Natl Cancer Inst 105 (12): 899-907, 2013.
-
Spiegler BJ, Kennedy K, Maze R, et al.: Comparison of long-term neurocognitive outcomes in young children with acute lymphoblastic leukemia treated with cranial radiation or high-dose or very high-dose intravenous methotrexate. J Clin Oncol 24 (24): 3858-64, 2006.
-
Campbell LK, Scaduto M, Sharp W, et al.: A meta-analysis of the neurocognitive sequelae of treatment for childhood acute lymphocytic leukemia. Pediatr Blood Cancer 49 (1): 65-73, 2007.
-
Cheung YT, Sabin ND, Reddick WE, et al.: Leukoencephalopathy and long-term neurobehavioural, neurocognitive, and brain imaging outcomes in survivors of childhood acute lymphoblastic leukaemia treated with chemotherapy: a longitudinal analysis. Lancet Haematol 3 (10): e456-e466, 2016.
-
Jansen NC, Kingma A, Schuitema A, et al.: Neuropsychological outcome in chemotherapy-only-treated children with acute lymphoblastic leukemia. J Clin Oncol 26 (18): 3025-30, 2008.
-
Iyer NS, Balsamo LM, Bracken MB, et al.: Chemotherapy-only treatment effects on long-term neurocognitive functioning in childhood ALL survivors: a review and meta-analysis. Blood 126 (3): 346-53, 2015.
-
Jacola LM, Krull KR, Pui CH, et al.: Longitudinal Assessment of Neurocognitive Outcomes in Survivors of Childhood Acute Lymphoblastic Leukemia Treated on a Contemporary Chemotherapy Protocol. J Clin Oncol 34 (11): 1239-47, 2016.
-
Espy KA, Moore IM, Kaufmann PM, et al.: Chemotherapeutic CNS prophylaxis and neuropsychologic change in children with acute lymphoblastic leukemia: a prospective study. J Pediatr Psychol 26 (1): 1-9, 2001 Jan-Feb.
-
Buizer AI, de Sonneville LM, Veerman AJ: Effects of chemotherapy on neurocognitive function in children with acute lymphoblastic leukemia: a critical review of the literature. Pediatr Blood Cancer 52 (4): 447-54, 2009.
-
Gandy K, Sapkota Y, Scoggins MA, et al.: Genetic variants, neurocognitive outcomes, and functional neuroimaging in survivors of childhood acute lymphoblastic leukemia. JNCI Cancer Spectr 7 (4): , 2023.
-
Cole PD, Finkelstein Y, Stevenson KE, et al.: Polymorphisms in Genes Related to Oxidative Stress Are Associated With Inferior Cognitive Function After Therapy for Childhood Acute Lymphoblastic Leukemia. J Clin Oncol 33 (19): 2205-11, 2015.
-
Jacola LM, Edelstein K, Liu W, et al.: Cognitive, behaviour, and academic functioning in adolescent and young adult survivors of childhood acute lymphoblastic leukaemia: a report from the Childhood Cancer Survivor Study. Lancet Psychiatry 3 (10): 965-972, 2016.
-
Jacola LM, Conklin HM, Krull KR, et al.: The Impact of Intensified CNS-Directed Therapy on Neurocognitive Outcomes in Survivors of Childhood Acute Lymphoblastic Leukemia Treated Without Cranial Irradiation. J Clin Oncol 40 (36): 4218-4227, 2022.
-
Krull KR, Cheung YT, Liu W, et al.: Chemotherapy Pharmacodynamics and Neuroimaging and Neurocognitive Outcomes in Long-Term Survivors of Childhood Acute Lymphoblastic Leukemia. J Clin Oncol 34 (22): 2644-53, 2016.
-
Halsey C, Buck G, Richards S, et al.: The impact of therapy for childhood acute lymphoblastic leukaemia on intelligence quotients; results of the risk-stratified randomized central nervous system treatment trial MRC UKALL XI. J Hematol Oncol 4: 42, 2011.
-
Kadan-Lottick NS, Brouwers P, Breiger D, et al.: A comparison of neurocognitive functioning in children previously randomized to dexamethasone or prednisone in the treatment of childhood acute lymphoblastic leukemia. Blood 114 (9): 1746-52, 2009.
-
Williams AM, Cheung YT, Hyun G, et al.: Childhood Neurotoxicity and Brain Resilience to Adverse Events during Adulthood. Ann Neurol 89 (3): 534-545, 2021.
-
Stefanski KJ, Anixt JS, Goodman P, et al.: Long-Term Neurocognitive and Psychosocial Outcomes After Acute Myeloid Leukemia: A Childhood Cancer Survivor Study Report. J Natl Cancer Inst 113 (4): 481-495, 2021.
-
Edelmann MN, Daryani VM, Bishop MW, et al.: Neurocognitive and Patient-Reported Outcomes in Adult Survivors of Childhood Osteosarcoma. JAMA Oncol 2 (2): 201-8, 2016.
-
Tonning Olsson I, Brinkman TM, Wang M, et al.: Neurocognitive and psychosocial outcomes in adult survivors of childhood soft-tissue sarcoma: A report from the St. Jude Lifetime Cohort. Cancer 126 (7): 1576-1584, 2020.
-
Tonning Olsson I, Brinkman TM, Hyun G, et al.: Neurocognitive outcomes in long-term survivors of Wilms tumor: a report from the St. Jude Lifetime Cohort. J Cancer Surviv 13 (4): 570-579, 2019.
-
Willard VW, Qaddoumi I, Chen S, et al.: Developmental and adaptive functioning in children with retinoblastoma: a longitudinal investigation. J Clin Oncol 32 (25): 2788-93, 2014.
-
Willard VW, Qaddoumi I, Pan H, et al.: Cognitive and Adaptive Functioning in Youth With Retinoblastoma: A Longitudinal Investigation Through 10 Years of Age. J Clin Oncol 39 (24): 2676-2684, 2021.
-
Brinkman TM, Merchant TE, Li Z, et al.: Cognitive function and social attainment in adult survivors of retinoblastoma: a report from the St. Jude Lifetime Cohort Study. Cancer 121 (1): 123-31, 2015.
-
Ehrhardt MJ, Sandlund JT, Zhang N, et al.: Late outcomes of adult survivors of childhood non-Hodgkin lymphoma: A report from the St. Jude Lifetime Cohort Study. Pediatr Blood Cancer 64 (6): , 2017.
-
Krull KR, Sabin ND, Reddick WE, et al.: Neurocognitive function and CNS integrity in adult survivors of childhood hodgkin lymphoma. J Clin Oncol 30 (29): 3618-24, 2012.
-
Hesko C, Liu W, Srivastava DK, et al.: Neurocognitive outcomes in adult survivors of neuroblastoma: A report from the Childhood Cancer Survivor Study. Cancer 129 (18): 2904-2914, 2023.
-
Wu NL, Phipps AI, Krull KR, et al.: Long-term patient-reported neurocognitive outcomes in adult survivors of hematopoietic cell transplant. Blood Adv 6 (14): 4347-4356, 2022.
-
Willard VW, Leung W, Huang Q, et al.: Cognitive outcome after pediatric stem-cell transplantation: impact of age and total-body irradiation. J Clin Oncol 32 (35): 3982-8, 2014.
-
Kenborg L, Winther JF, Linnet KM, et al.: Neurologic disorders in 4858 survivors of central nervous system tumors in childhood-an Adult Life after Childhood Cancer in Scandinavia (ALiCCS) study. Neuro Oncol 21 (1): 125-136, 2019.
-
Wells EM, Ullrich NJ, Seidel K, et al.: Longitudinal assessment of late-onset neurologic conditions in survivors of childhood central nervous system tumors: a Childhood Cancer Survivor Study report. Neuro Oncol 20 (1): 132-142, 2018.
-
Goldsby RE, Liu Q, Nathan PC, et al.: Late-occurring neurologic sequelae in adult survivors of childhood acute lymphoblastic leukemia: a report from the Childhood Cancer Survivor Study. J Clin Oncol 28 (2): 324-31, 2010.
-
Phillips NS, Khan RB, Li C, et al.: Seizures' impact on cognition and quality of life in childhood cancer survivors. Cancer 128 (1): 180-191, 2022.
-
Jain P, Gulati S, Seth R, et al.: Vincristine-induced neuropathy in childhood ALL (acute lymphoblastic leukemia) survivors: prevalence and electrophysiological characteristics. J Child Neurol 29 (7): 932-7, 2014.
-
Ness KK, Jones KE, Smith WA, et al.: Chemotherapy-related neuropathic symptoms and functional impairment in adult survivors of extracranial solid tumors of childhood: results from the St. Jude Lifetime Cohort Study. Arch Phys Med Rehabil 94 (8): 1451-7, 2013.
-
Kandula T, Farrar MA, Cohn RJ, et al.: Chemotherapy-Induced Peripheral Neuropathy in Long-term Survivors of Childhood Cancer: Clinical, Neurophysiological, Functional, and Patient-Reported Outcomes. JAMA Neurol 75 (8): 980-988, 2018.
-
Rodwin RL, Ross WL, Rotatori J, et al.: Newly identified chemotherapy-induced peripheral neuropathy in a childhood cancer survivorship clinic. Pediatr Blood Cancer 69 (3): e29550, 2022.
-
Varedi M, Lu L, Howell CR, et al.: Peripheral Neuropathy, Sensory Processing, and Balance in Survivors of Acute Lymphoblastic Leukemia. J Clin Oncol 36 (22): 2315-2322, 2018.
-
Gurney JG, Kadan-Lottick NS, Packer RJ, et al.: Endocrine and cardiovascular late effects among adult survivors of childhood brain tumors: Childhood Cancer Survivor Study. Cancer 97 (3): 663-73, 2003.
-
Ullrich NJ, Robertson R, Kinnamon DD, et al.: Moyamoya following cranial irradiation for primary brain tumors in children. Neurology 68 (12): 932-8, 2007.
-
Wang C, Roberts KB, Bindra RS, et al.: Delayed cerebral vasculopathy following cranial radiation therapy for pediatric tumors. Pediatr Neurol 50 (6): 549-56, 2014.
-
Mueller S, Fullerton HJ, Stratton K, et al.: Radiation, atherosclerotic risk factors, and stroke risk in survivors of pediatric cancer: a report from the Childhood Cancer Survivor Study. Int J Radiat Oncol Biol Phys 86 (4): 649-55, 2013.
-
Reulen RC, Guha J, Bright CJ, et al.: Risk of cerebrovascular disease among 13 457 five-year survivors of childhood cancer: A population-based cohort study. Int J Cancer 148 (3): 572-583, 2021.
-
Fullerton HJ, Stratton K, Mueller S, et al.: Recurrent stroke in childhood cancer survivors. Neurology 85 (12): 1056-64, 2015.
-
Waxer JF, Wong K, Modiri A, et al.: Risk of Cerebrovascular Events Among Childhood and Adolescent Patients Receiving Cranial Radiation Therapy: A PENTEC Normal Tissue Outcomes Comprehensive Review. Int J Radiat Oncol Biol Phys 119 (2): 417-430, 2024.
-
Khan RB, Merchant TE, Sadighi ZS, et al.: Prevalence, risk factors, and response to treatment for hypersomnia of central origin in survivors of childhood brain tumors. J Neurooncol 136 (2): 379-384, 2018.
-
van Kooten JAMC, Maurice-Stam H, Schouten AYN, et al.: High occurrence of sleep problems in survivors of a childhood brain tumor with neurocognitive complaints: The association with psychosocial and behavioral executive functioning. Pediatr Blood Cancer 66 (11): e27947, 2019.
-
Khan RB, Hudson MM, Ledet DS, et al.: Neurologic morbidity and quality of life in survivors of childhood acute lymphoblastic leukemia: a prospective cross-sectional study. J Cancer Surviv 8 (4): 688-96, 2014.
-
Khong PL, Leung LH, Fung AS, et al.: White matter anisotropy in post-treatment childhood cancer survivors: preliminary evidence of association with neurocognitive function. J Clin Oncol 24 (6): 884-90, 2006.
-
Zeller B, Tamnes CK, Kanellopoulos A, et al.: Reduced neuroanatomic volumes in long-term survivors of childhood acute lymphoblastic leukemia. J Clin Oncol 31 (17): 2078-85, 2013.
-
Faraci M, Morana G, Bagnasco F, et al.: Magnetic resonance imaging in childhood leukemia survivors treated with cranial radiotherapy: a cross sectional, single center study. Pediatr Blood Cancer 57 (2): 240-6, 2011.
-
Phillips NS, Hillenbrand CM, Mitrea BG, et al.: Cerebral microbleeds in adult survivors of childhood acute lymphoblastic leukemia treated with cranial radiation. Sci Rep 10 (1): 692, 2020.
-
Rodwin RL, Chen Y, Yasui Y, et al.: Longitudinal Evaluation of Neuromuscular Dysfunction in Long-term Survivors of Childhood Cancer: A Report from the Childhood Cancer Survivor Study. Cancer Epidemiol Biomarkers Prev 30 (8): 1536-1545, 2021.
-
Ness KK, Hudson MM, Jones KE, et al.: Effect of Temporal Changes in Therapeutic Exposure on Self-reported Health Status in Childhood Cancer Survivors. Ann Intern Med 166 (2): 89-98, 2017.
-
Ernst M, Hinz A, Brähler E, et al.: Quality of life after pediatric cancer: comparison of long-term childhood cancer survivors' quality of life with a representative general population sample and associations with physical health and risk indicators. Health Qual Life Outcomes 21 (1): 65, 2023.
-
Schulte F, Brinkman TM, Li C, et al.: Social adjustment in adolescent survivors of pediatric central nervous system tumors: A report from the Childhood Cancer Survivor Study. Cancer 124 (17): 3596-3608, 2018.
-
Hörnquist L, Rickardsson J, Lannering B, et al.: Altered self-perception in adult survivors treated for a CNS tumor in childhood or adolescence: population-based outcomes compared with the general population. Neuro Oncol 17 (5): 733-40, 2015.
-
Schulte F, Barrera M: Social competence in childhood brain tumor survivors: a comprehensive review. Support Care Cancer 18 (12): 1499-513, 2010.
-
Kunin-Batson A, Kadan-Lottick N, Zhu L, et al.: Predictors of independent living status in adult survivors of childhood cancer: a report from the Childhood Cancer Survivor Study. Pediatr Blood Cancer 57 (7): 1197-203, 2011.
-
Papini C, Willard VW, Gajjar A, et al.: Social cognition and adjustment in adult survivors of pediatric central nervous system tumors. Cancer 129 (19): 3064-3075, 2023.
-
Bonneau J, Berbis J, Michel G, et al.: Adolescence and Socioeconomic Factors: Key Factors in the Long-Term Impact of Leukemia on Scholastic Performance-A LEA Study. J Pediatr 205: 168-175.e2, 2019.
-
Drabbe C, Coenraadts ES, van Houdt WJ, et al.: Impaired social functioning in adolescent and young adult sarcoma survivors: Prevalence and risk factors. Cancer 129 (9): 1419-1431, 2023.
-
Ernst M, Brähler E, Wild PS, et al.: Risk factors for suicidal ideation in a large, registry-based sample of adult long-term childhood cancer survivors. J Affect Disord 265: 351-356, 2020.
-
Lubas MM, Mirzaei Salehabadi S, Lavecchia J, et al.: Suicidality among adult survivors of childhood cancer: A report from the St. Jude Lifetime Cohort Study. Cancer 126 (24): 5347-5355, 2020.
-
Brinkman TM, Zhang N, Recklitis CJ, et al.: Suicide ideation and associated mortality in adult survivors of childhood cancer. Cancer 120 (2): 271-7, 2014.
-
Gunnes MW, Lie RT, Bjørge T, et al.: Suicide and violent deaths in survivors of cancer in childhood, adolescence and young adulthood-A national cohort study. Int J Cancer 140 (3): 575-580, 2017.
-
Sun CL, Francisco L, Baker KS, et al.: Adverse psychological outcomes in long-term survivors of hematopoietic cell transplantation: a report from the Bone Marrow Transplant Survivor Study (BMTSS). Blood 118 (17): 4723-31, 2011.
-
Vuotto SC, Krull KR, Li C, et al.: Impact of chronic disease on emotional distress in adult survivors of childhood cancer: A report from the Childhood Cancer Survivor Study. Cancer 123 (3): 521-528, 2017.
-
Zheng DJ, Krull KR, Chen Y, et al.: Long-term psychological and educational outcomes for survivors of neuroblastoma: A report from the Childhood Cancer Survivor Study. Cancer 124 (15): 3220-3230, 2018.
-
Recklitis C, O'Leary T, Diller L: Utility of routine psychological screening in the childhood cancer survivor clinic. J Clin Oncol 21 (5): 787-92, 2003.
-
Hsu TW, Liang CS, Tsai SJ, et al.: Risk of Major Psychiatric Disorders Among Children and Adolescents Surviving Malignancies: A Nationwide Longitudinal Study. J Clin Oncol 41 (11): 2054-2066, 2023.
-
De R, Sutradhar R, Kurdyak P, et al.: Incidence and Predictors of Mental Health Outcomes Among Survivors of Adolescent and Young Adult Cancer: A Population-Based Study Using the IMPACT Cohort. J Clin Oncol 39 (9): 1010-1019, 2021.
-
Phipps S, Klosky JL, Long A, et al.: Posttraumatic stress and psychological growth in children with cancer: has the traumatic impact of cancer been overestimated? J Clin Oncol 32 (7): 641-6, 2014.
-
Stuber ML, Meeske KA, Leisenring W, et al.: Defining medical posttraumatic stress among young adult survivors in the Childhood Cancer Survivor Study. Gen Hosp Psychiatry 33 (4): 347-53, 2011 Jul-Aug.
-
Phipps S, Larson S, Long A, et al.: Adaptive style and symptoms of posttraumatic stress in children with cancer and their parents. J Pediatr Psychol 31 (3): 298-309, 2006.
-
Phipps S, Jurbergs N, Long A: Symptoms of post-traumatic stress in children with cancer: does personality trump health status? Psychooncology 18 (9): 992-1002, 2009.
-
Rourke MT, Hobbie WL, Schwartz L, et al.: Posttraumatic stress disorder (PTSD) in young adult survivors of childhood cancer. Pediatr Blood Cancer 49 (2): 177-82, 2007.
-
Schwartz L, Drotar D: Posttraumatic stress and related impairment in survivors of childhood cancer in early adulthood compared to healthy peers. J Pediatr Psychol 31 (4): 356-66, 2006.
-
Stuber ML, Meeske KA, Krull KR, et al.: Prevalence and predictors of posttraumatic stress disorder in adult survivors of childhood cancer. Pediatrics 125 (5): e1124-34, 2010.
-
Hobbie WL, Stuber M, Meeske K, et al.: Symptoms of posttraumatic stress in young adult survivors of childhood cancer. J Clin Oncol 18 (24): 4060-6, 2000.
-
Dieluweit U, Debatin KM, Grabow D, et al.: Social outcomes of long-term survivors of adolescent cancer. Psychooncology 19 (12): 1277-84, 2010.
-
Seitz DC, Hagmann D, Besier T, et al.: Life satisfaction in adult survivors of cancer during adolescence: what contributes to the latter satisfaction with life? Qual Life Res 20 (2): 225-36, 2011.
-
Tai E, Buchanan N, Townsend J, et al.: Health status of adolescent and young adult cancer survivors. Cancer 118 (19): 4884-91, 2012.
-
Schultz KA, Ness KK, Whitton J, et al.: Behavioral and social outcomes in adolescent survivors of childhood cancer: a report from the childhood cancer survivor study. J Clin Oncol 25 (24): 3649-56, 2007.
-
Prasad PK, Hardy KK, Zhang N, et al.: Psychosocial and Neurocognitive Outcomes in Adult Survivors of Adolescent and Early Young Adult Cancer: A Report From the Childhood Cancer Survivor Study. J Clin Oncol 33 (23): 2545-52, 2015.
-
Brinkman TM, Li C, Vannatta K, et al.: Behavioral, Social, and Emotional Symptom Comorbidities and Profiles in Adolescent Survivors of Childhood Cancer: A Report From the Childhood Cancer Survivor Study. J Clin Oncol 34 (28): 3417-25, 2016.
-
Karlson CW, Alberts NM, Liu W, et al.: Longitudinal pain and pain interference in long-term survivors of childhood cancer: A report from the Childhood Cancer Survivor Study. Cancer 126 (12): 2915-2923, 2020.
-
Tonning Olsson I, Alberts NM, Li C, et al.: Pain and functional outcomes in adult survivors of childhood cancer: A report from the St. Jude Lifetime Cohort study. Cancer 127 (10): 1679-1689, 2021.
-
Frederiksen LE, Erdmann F, Mader L, et al.: Psychiatric disorders in childhood cancer survivors in Denmark, Finland, and Sweden: a register-based cohort study from the SALiCCS research programme. Lancet Psychiatry 9 (1): 35-45, 2022.
Late Effects of the Digestive System
Dental
Overview
Chemotherapy, radiation therapy, and surgery can result in cosmetic and functional abnormalities of the oral cavity and dentition. The quality of current evidence regarding this outcome is limited by retrospective data collection, small sample size, cohort selection and participation bias, and heterogeneity in treatment approach, time since treatment, and method of ascertainment.
Oral and dental complications reported in childhood cancer survivors include the following:
- Abnormalities of tooth development.
- Salivary gland dysfunction.
- Abnormalities of craniofacial development.
- Osteoradionecrosis.
- Second cancers in the oral cavity.
Abnormalities of tooth development
Abnormalities of dental development reported in childhood cancer survivors include the following:[1,2,3,4,5,6,7,8,9,10,11,12]
- Absence of tooth development.
- Microdontia.
- Enamel hypoplasia.
- Root malformation.
- Hypodontia.
The prevalence of hypodontia has varied widely in series depending on age at diagnosis, treatment modality, and method of ascertainment.
Cancer treatments that have been associated with dental maldevelopment include the following:[3,10]
- Head and neck radiation therapy.
- Any chemotherapy.
- Hematopoietic stem cell transplant (HSCT).
Children younger than 5 years are at greatest risk of dental anomalies, including root agenesis, delayed eruption, enamel defects, and/or excessive caries related to disruption of ameloblast (enamel producing) and odontoblast (dentin producing) activity early in life.[3]
Key findings related to cancer treatment effect on tooth development include the following:
Radiation therapy
- Radiation directed at the oral cavity or surrounding structures increases the risk of dental anomalies because ameloblasts can be permanently damaged by doses as low as 10 Gy. In addition, salivary function is impacted by exposure to major and minor glands.[3,4,5]
- The most significant degree of tooth aplasia or delayed eruption occurs in younger children (aged <4 years) who are exposed to radiation doses of 20 Gy or higher.[13]
- Developing teeth may be irradiated when treating head and neck sarcomas, Hodgkin lymphoma, neuroblastoma, central nervous system leukemia, nasopharyngeal cancer, and brain tumors, or during total-body irradiation (TBI) for HSCT.
- Doses of 10 Gy to 40 Gy can cause root shortening or abnormal curvature, dwarfism, and hypocalcification.[14]
- Significant dental abnormalities, including mandibular or maxillary hypoplasia, increased caries, hypodontia, microdontia, root stunting, and xerostomia have been reported in more than 85% of survivors of head and neck rhabdomyosarcoma treated with radiation doses higher than 40 Gy.[4,15]
- In the St. Jude Life (SJLIFE) cohort, survivors who received radiation therapy that potentially exposed the oral cavity were more likely to report at least one dental health problem (odds ratio [OR], 1.48; 95% confidence interval [CI], 1.26–1.72) and at least one soft tissue abnormality (OR, 1.52; 95% CI, 1.25–1.84) after controlling for socioeconomic factors, age at last follow-up and diagnosis, other treatment exposures, and access to dental services.[12]
Chemotherapy
- Chemotherapy, especially exposure to alkylating agents, can affect tooth development.[3,5,6]
- Chemotherapy for the treatment of leukemia or neuroblastoma is associated with shortening and thinning of the premolar roots and enamel abnormalities.[8,16,17]
- Childhood Cancer Survivor Study (CCSS) investigators identified age younger than 5 years and increased exposure to cyclophosphamide as significant risk factors for developmental dental abnormalities in long-term survivors of childhood cancer.[3]
- In the SJLIFE study, survivors who received classic alkylating agents (OR, 1.6; 95% CI, 1.36–1.88) or anthracycline antibiotics (OR, 1.22; 95% CI, 1.04–1.42) were more likely to report at least one dental health problem after controlling for socioeconomic factors, age at last follow-up and diagnosis, other treatment exposures, and access to dental services.[12]
HSCT
- HSCT conditioning, especially regimens containing TBI, may result in tooth agenesis and root malformation.[1,2]
- Younger children who have not developed secondary teeth are most vulnerable.[1,2,5]
- Children who undergo HSCT with TBI may develop short V-shaped roots, microdontia, enamel hypoplasia, and/or premature apical closure.[1,2,7]
- Younger age at HSCT is associated with greater severity in dental maldevelopment and deficit in vertical growth of the lower face.[8]
- Dental abnormalities have been reported in patients who underwent HSCT without TBI, particularly in patients younger than 2 years at the time of the transplant.[17]
Salivary gland dysfunction
Xerostomia, the sensation of dry mouth, is a potential side effect after head and neck irradiation or HSCT that can severely impact quality of life.[18]
- Complications of reduced salivary secretion include the following:[18,19]
- Increased caries.
- Susceptibility to oral infections.
- Sleep disturbances.
- Difficulties with chewing, swallowing, and speaking.
- The prevalence of salivary gland dysfunction after cancer treatment varies based on measurement techniques (patient report vs. stimulated or unstimulated salivary secretion rates).[20]
- In general, the prevalence of self-reported persistent posttherapy xerostomia is low among childhood cancer survivors.
- In the CCSS, the prevalence of self-reported xerostomia in survivors was 2.8% compared with 0.3% in siblings, with an increased risk in survivors older than 30 years.[3]
Key findings related to cancer treatment effect on salivary gland function include the following:
Radiation therapy
- Salivary gland irradiation incidental to treatment of head and neck malignancies or Hodgkin lymphoma causes a qualitative and quantitative change in salivary flow, which can be reversible after doses of lower than 40 Gy but may be irreversible after higher doses, depending on whether sensitizing chemotherapy is also administered.[18]
- In a German study of 114 pediatric patients, the risk of acute and late xerostomia increased with parotid and submandibular gland dose. In general, grade 1 or higher xerostomia was seen in patients who received a maximum dose of higher than 20 Gy to the salivary glands. The odds of both acute and late xerostomia were higher in patients who received concurrent chemotherapy compared with those who received radiation therapy alone, with an OR for acute xerostomia of 3.64 (95% CI, 1.49–8.89) and for late xerostomia of 5.15 (95% CI, 1.20–22.15).[21]
- The Pediatric Normal Tissue Effects in the Clinic (PENTEC) initiative reported on late effects for childhood cancer survivors who were treated with radiation therapy for head and neck cancers.[22] Grades 1 or higher xerostomia were seen in patients who received a maximum dose of more than 20 Gy to the salivary glands. The odds of both acute and chronic xerostomia were greater in patients who had received concurrent chemotherapy and radiation therapy (acute xerostomia OR, 3.64; 95% CI, 1.49–8.89 and late xerostomia OR, 5.15; 95% CI, 1.20–22.15), compared with patients who were treated with radiation therapy alone. A mean parotid dose of 35 to 40 Gy was associated with a 32% risk of higher than grade 2 acute xerostomia and a 13% to 32% risk of chronic xerostomia.
HSCT
- HSCT recipients are at increased risk of salivary gland dysfunction related to transplant conditioning or graft-versus-host disease (GVHD).
- GVHD can cause hyposalivation and xerostomia with resultant dental disease.
- In a study of pediatric HSCT survivors, 60% of those exposed to a conditioning regimen with cyclophosphamide and 10 Gy single-dose TBI had decreased salivary secretion rates, compared with 26% of those who received cyclophosphamide and busulfan.[23]
- In another study, the prevalence of reduced salivary secretion did not differ among long-term survivors on the basis of the conditioning regimen (single-dose TBI, 47%; fractionated TBI, 47%; busulfan, 42%).[24]
Chemotherapy
- The association of chemotherapy alone with xerostomia remains controversial.[18]
- Only one study of pediatric patients demonstrated an excess risk (OR, 12.32; 95% CI, 2.1–74.4) of decreased stimulated saliva flow rates among patients treated with cyclophosphamide. However, an increase in dental caries was not noted and patient-reported xerostomia was not evaluated.[6]
Abnormalities of craniofacial development
- Craniofacial maldevelopment is a common adverse outcome among children treated with high-dose radiation therapy to the head and neck that frequently occurs in association with other oral cavity sequelae such as dental anomalies, xerostomia, and trismus.[4,25]
- The extent and severity of musculoskeletal disfigurement is related to age at treatment and radiation therapy volume and dose, with higher risk observed among younger patients and those who received 30 Gy or higher of radiation therapy.[25]
Other oral health complications
- Osteoradionecrosis of the jaw is a rare complication observed in childhood survivors treated with high-dose craniofacial radiation (>40 Gy), particularly after dental extractions in irradiated mandibles.[26,27]
- Remediation of cosmetic and functional abnormalities often requires multiple surgical interventions.
- The impact of infectious complications and alterations in the microflora during and after therapy is not known.[5]
Posttherapy management
- Some studies suggest that fluoride products or chlorhexidine rinses may be beneficial in patients who have undergone radiation therapy.[28]
- Dental caries are a problematic consequence of reduced salivary quality and flow. The use of topical fluoride can dramatically reduce the frequency of caries, and saliva substitutes and sialagogues can ameliorate sequelae such as xerostomia.[19]
For more information about oral complications in cancer patients, see Oral Complications of Cancer Therapies.
Table 5 summarizes oral and dental late effects and the related health screenings.
Table 5. Oral/Dental Late Effectsa
Predisposing Therapy |
Oral/Dental Effects |
Health Screening/Interventions |
CT = computed tomography; GVHD = graft-versus-host disease; HSCT = hematopoietic stem cell transplant; MRI = magnetic resonance imaging. |
a Adapted from theChildren's Oncology Group Long-Term Follow-Up Guidelines for Survivors of Childhood, Adolescent, and Young Adult Cancers. |
Any chemotherapy; radiation exposing oral cavity |
Dental developmental abnormalities; tooth/root agenesis; microdontia; root thinning/shortening; enamel dysplasia |
Dental evaluation and cleaning every 6 months |
Regular dental care including fluoride applications |
Consultation with orthodontist experienced in management of irradiated childhood cancer survivors |
Baseline Panorex x-ray before dental procedures to evaluate root development |
Radiation exposing oral cavity |
Malocclusion; temporomandibular joint dysfunction |
Dental evaluation and cleaning every 6 months |
Regular dental care including fluoride applications |
Consultation with orthodontist experienced in management of irradiated childhood cancer survivors |
Baseline Panorex x-ray before dental procedures to evaluate root development |
Referral to otolaryngologist for assistive devices for jaw opening |
Radiation exposing oral cavity; HSCT with history of chronic GVHD |
Xerostomia/salivary gland dysfunction; periodontal disease; dental caries; oral cancer (squamous cell carcinoma) |
Dental evaluation and cleaning every 6 months |
Supportive care with saliva substitutes, moistening agents, and sialogogues (pilocarpine) |
Regular dental care including fluoride applications |
Referral for biopsy of suspicious lesions |
Radiation exposing oral cavity (≥40 Gy) |
Osteoradionecrosis |
History: impaired or delayed healing after dental work |
Examination: persistent jaw pain, swelling or trismus |
Imaging studies (x-ray, CT scan and/or MRI) may assist in making diagnosis |
Surgical biopsy may be needed to confirm diagnosis |
Consider hyperbaric oxygen treatments |
Digestive Tract
Overview
The gastrointestinal (GI) tract is sensitive to the acute toxicities of chemotherapy, radiation therapy, and surgery. These important treatment modalities can also result in some long-term issues in a treatment- and dose-dependent manner.
Reports published about long-term GI tract outcomes are limited by retrospective data collection, small sample size, cohort selection and participation bias, heterogeneity in treatment approach, time since treatment, and method of ascertainment.
Treatment-related late effects include the following:
- Upper and lower digestive tract late effects associated with dose intensity of chemotherapy and/or abdominal radiation.
- Adhesions secondary to abdominal surgery predisposing to postoperative bowel obstruction.
Digestive tract–related late effects include the following:
- Esophageal dysmotility.
- Esophageal stricture.
- Gastroesophageal reflux.
- Gastritis, enteritis, or colitis.
- GI motility dysfunction (diarrhea, constipation, encopresis, bowel obstruction).
- Subsequent malignant neoplasms (SMNs).
Impact of cancer histology on GI outcomes
The abdomen is a relatively common location for several pediatric malignancies, including rhabdomyosarcoma, Wilms tumor, lymphoma, germ cell tumors, and neuroblastoma.
Intra-abdominal tumors often require multimodal therapy, occasionally necessitating resection of bowel, bowel-injuring chemotherapy, and/or radiation therapy. Thus, these tumors would be expected to be particularly prone to long-term digestive tract issues.
GI outcomes from selected cohort studies
Evidence (GI outcomes from selected cohort studies):
- Among 5-year childhood cancer survivors participating in the CCSS, the cumulative incidence of self-reported GI conditions was 37.6% at 20 years from cancer diagnosis (25.8% for upper GI complications and 15.5% for lower GI complications), representing an almost twofold excess risk of upper GI complications (relative risk [RR], 1.8; 95% CI, 1.6–2.0) and lower GI complications (RR, 1.9; 95% CI, 1.7–2.2), compared with sibling controls.[29]
Factors predicting higher risk of specific GI complications include the following:
- Older age at diagnosis.
- Intensified therapy (anthracyclines for upper GI complications and alkylating agents for lower GI complications).
- Abdominal radiation therapy.
- Abdominal surgery.
Radiation-related GI injury
- Late radiation injury to the digestive tract is attributable to vascular injury.
- Necrosis, ulceration, stenosis, or perforation can occur and are characterized by malabsorption, pain, and recurrent episodes of bowel obstruction, as well as perforation and infection.[30,31,32]
- In general, fractionated radiation doses of 20 Gy to 30 Gy can be delivered to the small bowel without significant long-term morbidity.[33]
- Doses higher than 40 Gy are associated with a greater risk of bowel obstruction or chronic enterocolitis.[33]
- Sensitizing chemotherapeutic agents such as dactinomycin or anthracyclines can increase this risk.
A limited number of reports describe GI complications in pediatric patients with genitourinary solid tumors treated with radiation therapy:
- The CCSS evaluated the long-term morbidity among unilateral, nonsyndromic Wilms tumor survivors (n = 2,008) according to conventional treatment regimens. All patients underwent unilateral nephrectomy.[34]
- The 35-year cumulative incidence for intestinal obstruction was 8.1% (95% CI, 6.6%–9.6%).
- Relative to siblings, survivors treated with vincristine and actinomycin D (VA) demonstrated an elevated risk of intestinal obstruction (RR, 9.4). The risk with VA treatment was lower than for treatment groups who received more intensive therapy (RR range, 15.4–46.8).
- The rate of intestinal obstruction was highest among survivors who received more than 20 Gy of whole abdomen or flank radiation therapy.
- The CCSS evaluated the incidence and risk of late-occurring intestinal obstruction requiring surgery in 12,316 5-year survivors (2,002 with and 10,314 without abdominopelvic tumors) and 4,023 siblings.[35]
- The most common diagnoses among survivors with abdominopelvic tumors were Wilms tumors and neuroblastomas but also included soft tissue sarcomas, lymphomas, and bone tumors.
- The cumulative incidence of late intestinal obstruction requiring surgery at 35 years was 5.8% among survivors with abdominopelvic tumors, 1.0% among those without abdominopelvic tumors, and 0.3% among siblings.
- Elevated risk of intestinal obstruction requiring surgery was associated with presence of an abdominopelvic tumor (adjusted rate ratio [ARR], 3.6; P < .001) and exposure to abdominal or pelvic radiation therapy within 5 years of cancer diagnosis (ARR, 2.4; P < .001).
- Among survivors of abdominopelvic tumors, the median time from diagnosis to the first late intestinal obstruction requiring surgery was 12 years (range, 8–19 years).
- Lymphoma resulted in the highest cumulative incidence of late-occurring intestinal obstruction that required surgery (7.2% at 35 years after diagnosis).
- The CCSS evaluated late-onset anorectal disease in cohort members:[36]
- Among survivors, pelvic radiation therapy higher than 30 Gy within 5 years of cancer diagnosis was associated with late-onset anorectal disease (ARR for 30–49.9 Gy vs. none, 1.6; ARR for ≥50 Gy vs. none, 5.4).
- The most frequent anorectal disease reported was fistula, followed by stricture and anorectal SMN.
- Late-onset anorectal disease was associated with psychological impairment in all domains, as characterized by increased emotional distress and impaired quality of life.
- Reports from the Intergroup Rhabdomyosarcoma Study evaluating GI toxicity in long-term survivors of genitourinary rhabdomyosarcoma infrequently observed abnormalities of the irradiated bowel.[37,38,39]
- Radiation-related complications occurred in approximately 10% of long-term survivors of paratesticular and bladder/prostate rhabdomyosarcoma and included intraperitoneal adhesions with bowel obstruction, chronic diarrhea, and stricture or enteric fistula formation.
- The CCSS evaluated the prevalence of esophageal stricture and identified associated clinical and treatment risk factors among 17,121 5-year childhood cancer survivors and 3,400 siblings.[40]
- The prevalence of esophageal stricture among survivors was 2.0% (95% CI, 1.8%–2.2%), representing a 7.6-fold increased risk compared with siblings.
- Factors significantly associated with risk of esophageal stricture included a diagnosis of Hodgkin lymphoma, greater chest radiation dose, younger age at cancer diagnosis, platinum chemotherapy exposure, and undergoing an HSCT.
Table 6 summarizes digestive tract late effects and the related health screenings.
Table 6. Digestive Tract Late Effectsa
Predisposing Therapy |
Gastrointestinal Effects |
Health Screening/Interventions |
GVHD = graft-versus-host disease; HSCT = hematopoietic stem cell transplant; KUB = kidneys, ureter, bladder (plain abdominal radiograph). |
a Adapted from theChildren's Oncology Group Long-Term Follow-Up Guidelines for Survivors of Childhood, Adolescent, and Young Adult Cancers. |
Radiation exposing esophagus; HSCT with any history of chronic GVHD |
Gastroesophageal reflux; esophageal dysmotility; esophageal stricture |
History: dysphagia, heart burn |
Esophageal dilation, medical management, antireflux surgery |
Radiation exposing bowel |
Chronic enterocolitis; fistula; strictures |
History: nausea, vomiting, abdominal pain, diarrhea |
Serum protein and albumin levels yearly in patients with chronic diarrhea or fistula; gastroenterology consultation |
Surgical and/or gastroenterology consultation for symptomatic patients |
Radiation exposing bowel; laparotomy |
Bowel obstruction |
History: abdominal pain, distention, vomiting, constipation |
Examination: tenderness, abdominal guarding, distension (acute episode) |
Clinical evaluation in patients with symptoms of obstruction |
Surgical consultation in patients unresponsive to medical management |
Pelvic surgery; cystectomy |
Fecal incontinence |
History: chronic constipation, fecal soiling |
Rectal examination |
Hepatobiliary
Overview
Hepatic complications resulting from childhood cancer therapy are observed primarily as acute treatment toxicities.[41] Because many chemotherapy agents and radiation are hepatotoxic, transient liver function anomalies are common during therapy. Severe acute hepatic complications rarely occur. Survivors of childhood cancer can occasionally exhibit long-standing hepatic injury.[42]
Some general concepts regarding hepatotoxicity related to childhood cancer include the following:
- The risk of long-term hepatotoxicity is not well defined.
- Children with primary liver tumors requiring significant liver resection, or even transplant, are at higher risk of liver injury.
- Children receiving radiation therapy to the liver are at higher risk of liver injury.
- Children undergoing bone marrow transplant are at higher risk of liver injury.
Certain factors, including the type of chemotherapy, the dose and extent of radiation exposure, the influence of surgical interventions, and the evolving impact of viral hepatitis and/or other infectious complication, need additional attention in future studies.
Types of hepatobiliary late effects
Asymptomatic elevation of liver enzymes is the most common hepatobiliary complication.
-
Asymptomatic elevation of liver enzymes. Liver injury related to treatment for childhood cancer is often asymptomatic and indolent in course. While elevated serum alanine aminotransferase (ALT), aspartate aminotransferase (AST) and gamma glutamyltransferase (GGT) levels can reflect transient acute liver injury during chemotherapy, they are not predictive of late hepatic dysfunction or cirrhosis.
- Dutch investigators observed hepatobiliary dysfunction in 8.7% of 1,362 long-term survivors (median follow-up, 12.4 years since diagnosis) evaluated by ALT for hepatocellular injury and GGT for biliary tract injury. Cases with a history of viral hepatitis and a history of veno-occlusive disease were excluded.[43]
- Predictors for elevated ALT and GGT by multivariable analysis included treatment with radiation therapy involving the liver, higher body mass index (BMI), higher alcohol intake, and longer follow-up time; older age at diagnosis was only significantly associated with elevated GGT levels.
- An SJLIFE study evaluated prevalence of and risk factors for elevated ALT among 2,751 adult survivors of childhood cancer (median age, 31.4 years; median elapsed time from diagnosis, 23.2 years).[44]
- ALT greater than sex-specific upper limits of normal was prevalent in 41.3% of survivors; however, the prevalence of grade 3 or 4 hepatic injury was infrequent (<1%).
- Independent risk factors for elevated ALT included non-Hispanic White race and ethnicity, older age at evaluation, being overweight or affected by obesity, presence of metabolic syndrome, current treatment with a statin, hepatitis C infection, previous treatment with busulfan or thioguanine, history of hepatic surgery, and the percentage of liver treated with 10 Gy, 15 Gy, 20 Gy, or higher doses.
Less commonly reported hepatobiliary complications include the following:[45]
Cholelithiasis
- In limited studies, an increased risk of cholelithiasis has been linked to ileal conduit, parenteral nutrition, abdominal surgery, abdominal radiation therapy, and HSCT.[46,47]
- The cumulative incidence of late (occurring 5 or more years after cancer diagnosis) cholecystectomy among 25,549 CCSS participants diagnosed between 1970 and 1999 (median follow-up, 21.9 years) was 7.2% compared with 6.6% in a sibling control group (rate ratio, 1.3; 95% CI, 1.1–1.5).[48]
- Independent risk factors for late cholecystectomy include attained age, female sex, increasing BMI, exposure to high-dose (>750 mg/m2) platinum chemotherapy (rate ratio, 2.6; 95% CI, 1.5–4.5), vinca alkaloid chemotherapy (rate ratio, 1.4; 95% CI, 1.1–1.8) or TBI (rate ratio, 2.2; 95% CI, 1.2–2.4).[48]
Focal nodular hyperplasia
- Lesions made up of regenerating liver called focal nodular hyperplasia (FNH) have been incidentally noted on screening imaging studies after chemotherapy or HSCT.[49,50]
- FNH is thought to represent iatrogenic benign manifestations of vascular damage and have been associated with veno-occlusive disease, high-dose alkylating agents (e.g., busulfan and melphalan), and liver irradiation.[49]
- The prevalence of FNH is unknown; while noted at less than 1% in some papers,[50] this is likely an underestimate.
- In one study of patients who were followed by magnetic resonance imaging (MRI) after transplant to assess liver iron stores, the cumulative incidence of FNH was 35% at 150 months posttransplant.[49]
- FNH can mimic metastatic or subsequent tumors, but MRI imaging has a characteristic pattern and is generally diagnostic.[49]
- Biopsy or resection is usually unnecessary unless the lesions grow or patients have worrisome symptoms.[49]
Nodular regenerative hyperplasia
- Nodular regenerative hyperplasia is a rare condition characterized by the development of multiple monoacinar regenerative hepatic nodules and mild fibrosis.[45]
- The pathogenesis of nodular regenerative hyperplasia is not well established but may represent a nonspecific tissue adaptation to heterogeneous hepatic blood flow.[51]
- Nodular regenerative hyperplasia has rarely been observed in survivors of childhood cancer treated with chemotherapy, with or without liver irradiation.[52,53]
- Biopsy may be necessary to distinguish nodular regenerative hyperplasia from a subsequent malignancy.[53]
Microvesicular fatty change
- Histological evidence of fatty infiltration (93%) and siderosis (up to 70%) was observed in children with acute lymphoblastic leukemia (ALL) who recently completed intensified therapy. Fibrosis developed in 11% and was associated with higher serum low-density lipoprotein (LDL) cholesterol.[54]
- Fatty liver with insulin resistance has also been reported to develop more frequently in long-term childhood cancer survivors treated with cranial radiation and TBI therapy with allogeneic HSCT without overweight or obesity.[55]
- Prospective studies are needed to define whether acute posttherapy fatty liver change contributes to the development of late steatohepatitis or the metabolic syndrome in this population.
Acquired hemochromatosis
- Red blood cell transfusion can result in an accumulation of excess iron caused by the disruption of the homeostasis of iron storage and distribution when exogenous iron is loaded into organs.
- Transfusional iron overload has been reported in pediatric oncology patients, but its prevalence, organ distribution, and severity remain incompletely characterized.
- MRI has emerged as an accurate, noninvasive means for measuring iron in multiple organ systems.[56,57]
- In a cross-sectional study of 75 patients (4.4 years of median follow-up time; 4.9 years since last transfusion), MRI iron concentrations were elevated in the liver (49.3%) and pancreas (26.4%), but not in the heart.[56]
- In a multivariable analysis, cumulative packed red blood cell volume and older age at diagnosis predicted elevated liver iron concentration.[56]
- Receipt of allogeneic transplant is a significant risk factor for transfusional iron overload.[58]
Treatment-related risk factors for hepatobiliary late effects
The type and intensity of previous therapy influences risk for late-occurring hepatobiliary effects. In addition to the risk of treatment-related toxicity, recipients of HSCT frequently experience chronic liver dysfunction related to microvascular, immunologic, infectious, metabolic, and other toxic etiologies.
Key findings related to cancer treatment effect on hepatobiliary complications include the following:
Chemotherapy
- Chemotherapeutic agents with established hepatotoxic potential include antimetabolite agents like 6-mercaptopurine, 6-thioguanine, methotrexate, and rarely, dactinomycin.
- Veno-occlusive disease/sinusoidal obstruction syndrome (VOD/SOS) and cholestatic disease have been observed after thiopurine administration, especially 6-thioguanine.[59]
- Progressive fibrosis and portal hypertension have been reported in a subset of children who developed VOD/SOS after treatment with 6-thioguanine.[59]
- Acute, dose-related, reversible VOD/SOS has been observed in children treated with dactinomycin for pediatric solid tumors.[60,61]
- In the transplant setting, VOD/SOS has also been observed after conditioning regimens that have included cyclophosphamide/TBI, busulfan/cyclophosphamide, and carmustine/cyclophosphamide/etoposide.[62] High-dose cyclophosphamide, common to all of these regimens, is speculated to be a potential causative factor.
Radiation therapy
- Acute radiation-induced liver disease also causes endothelial cell injury that is characteristic of VOD/SOS.[63]
- In adults, the whole liver has tolerance up to 30 Gy to 35 Gy with conventional fractionation, the prevalence of radiation-induced liver disease varies from 6% to 66% based on the volume of liver involved and on hepatic reserve.[63,64]
- Radiation hepatopathy after contemporary treatment appears to be uncommon in long-term survivors without predisposing conditions, such as viral hepatitis or iron overload.[65]
- The risk of injury in children increases with radiation dose, hepatic volume, younger age at treatment, previous partial hepatectomy, and concomitant use of radiomimetic chemotherapy, such as dactinomycin and doxorubicin.[66,67,68,69]
- Survivors who received radiation doses of 40 Gy to at least one-third of the liver volume, doses of 30 Gy or higher to the whole abdomen, or an upper abdominal field involving the entire liver are at highest risk for hepatic dysfunction.[42]
- A PENTEC comprehensive review was performed to develop models to inform dose constraints for radiation-associated hepatic toxicity.[70]
- In a model developed to calculate the risk of developing SOS after whole-liver radiation therapy, radiation dose and receipt of nonalkylating chemotherapy were significant. Age younger than 20 years at the time of radiation therapy was borderline significant.
- The predicted risk of SOS was 2% with zero radiation therapy dose, 6.1% after treatment with 10 Gy, and 14.5% after treatment with 20 Gy to the whole liver.
- Patients with Wilms tumor treated with right flank radiation therapy (estimated dose, 6.54 ± 2.50 Gy) had a higher observed rate of SOS than patients who received left flank radiation therapy (estimated dose, 2.16 ± 1.15 Gy).
- Data were sparse regarding rates of late liver injury after radiation therapy, which suggests low rates of severe toxicity after treatment for common pediatric malignancies.
HSCT
- Chronic liver dysfunction in patients after HSCT is multifactorial in etiology with the most common etiologies including iron overload, chronic GVHD, and viral hepatitis.[71]
- Patients with chronic GVHD of the GI tract who exhibit an elevated bilirubin have a worse prognosis and quality of life.[72]
- While chronic liver dysfunction may be seen in more than one-half of long-term HSCT survivors, and the course of the disease appears to be indolent, continued follow-up is needed to establish its long-term impact on survivor health.[73]
Infectious risk factors for hepatobiliary late effects
Viral hepatitis B and C may complicate the treatment course of childhood cancer and result in chronic hepatic dysfunction.
- The incidence of transfusion-related hepatitis C in childhood cancer survivors has ranged from 5% to 50% depending on the geographic location of the reporting center.[74,75,76,77,78,79,80]
- Chronic hepatitis predisposes the childhood cancer survivor to cirrhosis, end-stage liver disease, and hepatocellular carcinoma.[78,79]
- Concurrent infection with hepatitis B and C in combination or in co-occurrence with other hepatotrophic viruses accelerates the progression of liver disease.[75,77]
- Because most patients received some type of blood product during childhood cancer treatment and many are unaware of their transfusion history, screening on the basis of date of diagnosis/treatment is recommended.[81]
- All survivors of childhood cancer who received treatment before 1972 should be screened for hepatitis B, and those who received treatment before 1993 should be screened for hepatitis C and referred for discussion of treatment options if screening results are positive.[81]
Posttherapy management
Survivors with liver dysfunction should be counseled regarding risk-reduction methods to prevent hepatic injury.
- Standard recommendations include maintenance of a healthy body weight, abstinence from alcohol use, and immunization against hepatitis A and B viruses.[42]
- In patients with chronic hepatitis, precautions to reduce viral transmission to household and sexual contacts should also be reviewed.
Table 7 summarizes hepatobiliary late effects and the related health screenings.
Table 7. Hepatobiliary Late Effectsa
Predisposing Therapy |
Hepatic Effects |
Health Screening/Interventions |
ALT = alanine aminotransferase; AST = aspartate aminotransferase; HSCT = hematopoietic stem cell transplant. |
a Adapted from theChildren's Oncology Group Long-Term Follow-Up Guidelines for Survivors of Childhood, Adolescent, and Young Adult Cancers. |
Methotrexate; mercaptopurine/thioguanine; HSCT |
Hepatic dysfunction |
Laboratory tests: ALT, AST, bilirubin levels |
Ferritin in those treated with HSCT |
Mercaptopurine/thioguanine; HSCT |
Veno-occlusive disease/sinusoidal obstructive syndrome |
Examination: scleral icterus, jaundice, ascites, hepatomegaly, splenomegaly |
Laboratory tests: ALT, AST, bilirubin, platelet levels |
Ferritin in those treated with HSCT |
Radiation exposing liver/biliary tract; HSCT |
Hepatic fibrosis/cirrhosis; focal nodular hyperplasia |
Examination: jaundice, spider angiomas, palmar erythema, xanthomata, hepatomegaly, splenomegaly |
Laboratory tests: ALT, AST, bilirubin levels |
Ferritin in those treated with HSCT |
Prothrombin time for evaluation of hepatic synthetic function in patients with abnormal liver screening tests |
Screen for viral hepatitis in patients with persistently abnormal liver function or any patient transfused before 1993 |
Gastroenterology/hepatology consultation in patients with persistent liver dysfunction |
Hepatitis A and B immunizations in patients lacking immunity |
Consider phlebotomy and chelation therapy for iron overload |
Radiation exposing liver/biliary tract |
Cholelithiasis |
History: colicky abdominal pain related to fatty food intake, excessive flatulence |
Examination: right upper quadrant or epigastric tenderness (acute episode) |
Consider gallbladder ultrasonography in patients with chronic abdominal pain |
Pancreas
The pancreas has been thought to be relatively radioresistant because of a paucity of information about late pancreatic-related effects. However, children and young adults treated with TBI or abdominal irradiation are known to have an increased risk of insulin resistance and diabetes mellitus.[82,83,84]
While corticosteroids and asparaginase are associated with acute toxicity to the pancreas, late sequelae in the form of exocrine or endocrine pancreatic function for those who sustain acute injury have not been reported.
Evidence (risk of diabetes mellitus):
- The prevalence of prediabetes among survivors of childhood cancer, risk factors for progression to diabetes, and association between prediabetes and late cardiovascular events and chronic kidney disease were investigated using the SJLIFE cohort.[85]
- The prevalence of prediabetes (defined as fasting plasma glucose level of 100–125 mg/dL or hemoglobin A1c of 5.7%–6.4%) was assessed in 3,529 adult survivors of childhood cancer. The prevalence of prediabetes in survivors (median age, 30 years) was 29.2%, and the prevalence of diabetes was 6.5%.
- By age 40 to 49 years, the prevalence of prediabetes was 45.5%, and in each age strata, survivors had a significantly higher prevalence of prediabetes and diabetes than controls (P < .0025). Among the 695 survivors with prediabetes who underwent longitudinal follow-up, 10% progressed to diabetes.
- Radiation exposure to the pancreatic tail was the only treatment that significantly increased the risk of prediabetes, independent of age, sex, race, BMI, physical activity, and other therapy exposures.
- Compared with survivors with normal glucose control, adjusting for relevant treatment exposures, survivors with prediabetes were at an increased risk of future myocardial infarction (hazard ratio [HR], 2.4) and chronic kidney disease (HR, 2.9), while survivors with diabetes were also at an increased risk of future cardiomyopathy (HR, 3.8) and stroke (HR, 3.4).
- CCSS investigators evaluated the risk of diabetes mellitus among 20,762 5-year childhood cancer survivors and 4,853 siblings.[86]
- Survivors exposed to abdominal radiation (n = 4,568) were almost three times more likely to develop diabetes than were siblings and 1.6 times more likely than survivors who were not exposed to abdominal radiation.
- Among survivors treated with abdominal radiation therapy, multivariable modeling identified independent risk factors for developing diabetes, which included older attained age, higher BMI, and increasing dose to the pancreatic tail.
- A significant interaction was also identified between younger age (<10 years) at cancer diagnosis and higher mean pancreatic tail dose.
- SJLIFE study investigators evaluated the prevalence of and risk factors for diabetes mellitus among 1,044 adult survivors of childhood ALL (mean age, 34 years) who were clinically assessed more than 10 years after treatment and 368 community controls (mean age, 35 years).[87]
- Type 2 diabetes mellitus was prevalent in 7.5% of survivors and 3.8% of controls.
- Independent risk factors for developing diabetes among survivors included older age (OR, 1.05 for each additional year), BMI of 30 kg/m2 or higher (OR, 7.4), and history of drug-induced hyperglycemia during therapy (OR, 4.67).
- A retrospective cohort study, based on self-reports of 2,520 5-year survivors of childhood cancer treated in France and the United Kingdom, investigated the relationship between radiation dose to the pancreas and risk of a subsequent diabetes mellitus diagnosis.[88]
- Sixty-five cases of diabetes mellitus were validated; the risk increased with radiation therapy to the tail of the pancreas, where the islets of Langerhans are concentrated. Risk increased up to 20 to 29 Gy and then plateaued. The estimated RR at 1 Gy was 1.61.
- Radiation dose to other parts of the pancreas did not have a significant effect.
- Compared with patients who did not receive radiation therapy, the RR of diabetes mellitus was 11.5 in patients who received more than 10 Gy to the pancreas.
- Children younger than 2 years at the time of radiation therapy were more sensitive than were older patients (RR at 1 Gy was 2.1 for the young age group vs. 1.4 for older patients).
- For the 511 patients who received more than 10 Gy, the cumulative incidence of diabetes mellitus was 16%.
- Another study evaluated the risk of diabetes mellitus in 2,264 5-year survivors of Hodgkin lymphoma (42% younger than 25 years at diagnosis) after a median follow-up of 21.5 years.[89]
- The cumulative incidence of diabetes mellitus was 8.3% (95% CI, 6.9%–9.8%) for the overall cohort and 14.2% (95% CI, 10.7%–18.3%) for those treated with more than 36 Gy para-aortic radiation.
- Survivors treated with more than 36 Gy of radiation to the para-aortic lymph nodes and spleen had a 2.3-fold increased risk of diabetes mellitus compared with those who did not receive radiation therapy.
- The risk of diabetes mellitus increased with higher doses to the pancreatic tail.
For digestive system late effects information, including risk factors, evaluation, and health counseling, see the Children's Oncology Group Long-Term Follow-Up Guidelines for Survivors of Childhood, Adolescent, and Young Adult Cancers.
References:
-
Hölttä P, Alaluusua S, Saarinen-Pihkala UM, et al.: Agenesis and microdontia of permanent teeth as late adverse effects after stem cell transplantation in young children. Cancer 103 (1): 181-90, 2005.
-
Hölttä P, Hovi L, Saarinen-Pihkala UM, et al.: Disturbed root development of permanent teeth after pediatric stem cell transplantation. Dental root development after SCT. Cancer 103 (7): 1484-93, 2005.
-
Kaste SC, Goodman P, Leisenring W, et al.: Impact of radiation and chemotherapy on risk of dental abnormalities: a report from the Childhood Cancer Survivor Study. Cancer 115 (24): 5817-27, 2009.
-
Paulino AC, Simon JH, Zhen W, et al.: Long-term effects in children treated with radiotherapy for head and neck rhabdomyosarcoma. Int J Radiat Oncol Biol Phys 48 (5): 1489-95, 2000.
-
Maciel JC, de Castro CG, Brunetto AL, et al.: Oral health and dental anomalies in patients treated for leukemia in childhood and adolescence. Pediatr Blood Cancer 53 (3): 361-5, 2009.
-
Hsieh SG, Hibbert S, Shaw P, et al.: Association of cyclophosphamide use with dental developmental defects and salivary gland dysfunction in recipients of childhood antineoplastic therapy. Cancer 117 (10): 2219-27, 2011.
-
Dahllöf G: Oral and dental late effects after pediatric stem cell transplantation. Biol Blood Marrow Transplant 14 (1 Suppl 1): 81-3, 2008.
-
Hölttä P, Alaluusua S, Saarinen-Pihkala UM, et al.: Long-term adverse effects on dentition in children with poor-risk neuroblastoma treated with high-dose chemotherapy and autologous stem cell transplantation with or without total body irradiation. Bone Marrow Transplant 29 (2): 121-7, 2002.
-
Effinger KE, Migliorati CA, Hudson MM, et al.: Oral and dental late effects in survivors of childhood cancer: a Children's Oncology Group report. Support Care Cancer 22 (7): 2009-19, 2014.
-
Kang CM, Hahn SM, Kim HS, et al.: Clinical Risk Factors Influencing Dental Developmental Disturbances in Childhood Cancer Survivors. Cancer Res Treat 50 (3): 926-935, 2018.
-
Çetiner D, Çetiner S, Uraz A, et al.: Oral and dental alterations and growth disruption following chemotherapy in long-term survivors of childhood malignancies. Support Care Cancer 27 (5): 1891-1899, 2019.
-
Patni T, Lee CT, Li Y, et al.: Factors for poor oral health in long-term childhood cancer survivors. BMC Oral Health 23 (1): 73, 2023.
-
Thompson RF, Schneider RA, Albertini F, et al.: Dose to the developing dentition during therapeutic irradiation: organ at risk determination and clinical implications. Int J Radiat Oncol Biol Phys 86 (1): 108-13, 2013.
-
Maguire A, Craft AW, Evans RG, et al.: The long-term effects of treatment on the dental condition of children surviving malignant disease. Cancer 60 (10): 2570-5, 1987.
-
Paulino AC: Role of radiation therapy in parameningeal rhabdomyosarcoma. Cancer Invest 17 (3): 223-30, 1999.
-
Kaste SC, Hopkins KP, Jones D, et al.: Dental abnormalities in children treated for acute lymphoblastic leukemia. Leukemia 11 (6): 792-6, 1997.
-
Elzembely MM, Dahlberg AE, Pinto N, et al.: Late effects in high-risk neuroblastoma survivors treated with high-dose chemotherapy and stem cell rescue. Pediatr Blood Cancer 66 (1): e27421, 2019.
-
Jensen SB, Pedersen AM, Vissink A, et al.: A systematic review of salivary gland hypofunction and xerostomia induced by cancer therapies: prevalence, severity and impact on quality of life. Support Care Cancer 18 (8): 1039-60, 2010.
-
Jensen SB, Pedersen AM, Vissink A, et al.: A systematic review of salivary gland hypofunction and xerostomia induced by cancer therapies: management strategies and economic impact. Support Care Cancer 18 (8): 1061-79, 2010.
-
Garming Legert K, Remberger M, Ringdèn O, et al.: Salivary secretion in children after fractionated or single-dose TBI. Bone Marrow Transplant 47 (3): 404-10, 2012.
-
Bölling T, Weege J, Eich HT, et al.: Acute and late side effects to salivary glands and oral mucosa after head and neck radiotherapy in children and adolescents. Results of the "Registry for the evaluation of side effects after radiotherapy in childhood and adolescence". Head Neck 37 (8): 1137-41, 2015.
-
Milgrom SA, van Luijk P, Pino R, et al.: Salivary and Dental Complications in Childhood Cancer Survivors Treated With Radiation Therapy to the Head and Neck: A PENTEC Comprehensive Review. Int J Radiat Oncol Biol Phys 119 (2): 467-481, 2024.
-
Dahllöf G, Wondimu B, Barr-Agholme M, et al.: Xerostomia in children and adolescents after stem cell transplantation conditioned with total body irradiation or busulfan. Oral Oncol 47 (9): 915-9, 2011.
-
Garming-Legert K, Remberger M, Ringdén O, et al.: Long-term salivary function after conditioning with busulfan, fractionated or single-dose TBI. Oral Dis 17 (7): 670-6, 2011.
-
Gevorgyan A, La Scala GC, Neligan PC, et al.: Radiation-induced craniofacial bone growth disturbances. J Craniofac Surg 18 (5): 1001-7, 2007.
-
Sulaiman F, Huryn JM, Zlotolow IM: Dental extractions in the irradiated head and neck patient: a retrospective analysis of Memorial Sloan-Kettering Cancer Center protocols, criteria, and end results. J Oral Maxillofac Surg 61 (10): 1123-31, 2003.
-
Vissink A, Jansma J, Spijkervet FK, et al.: Oral sequelae of head and neck radiotherapy. Crit Rev Oral Biol Med 14 (3): 199-212, 2003.
-
Hong CH, Napeñas JJ, Hodgson BD, et al.: A systematic review of dental disease in patients undergoing cancer therapy. Support Care Cancer 18 (8): 1007-21, 2010.
-
Goldsby R, Chen Y, Raber S, et al.: Survivors of childhood cancer have increased risk of gastrointestinal complications later in life. Gastroenterology 140 (5): 1464-71.e1, 2011.
-
Bölling T, Willich N, Ernst I: Late effects of abdominal irradiation in children: a review of the literature. Anticancer Res 30 (1): 227-31, 2010.
-
Churnratanakul S, Wirzba B, Lam T, et al.: Radiation and the small intestine. Future perspectives for preventive therapy. Dig Dis 8 (1): 45-60, 1990.
-
Sher ME, Bauer J: Radiation-induced enteropathy. Am J Gastroenterol 85 (2): 121-8, 1990.
-
Emami B, Lyman J, Brown A, et al.: Tolerance of normal tissue to therapeutic irradiation. Int J Radiat Oncol Biol Phys 21 (1): 109-22, 1991.
-
Weil BR, Murphy AJ, Liu Q, et al.: Late Health Outcomes Among Survivors of Wilms Tumor Diagnosed Over Three Decades: A Report From the Childhood Cancer Survivor Study. J Clin Oncol 41 (14): 2638-2650, 2023.
-
Madenci AL, Fisher S, Diller LR, et al.: Intestinal Obstruction in Survivors of Childhood Cancer: A Report From the Childhood Cancer Survivor Study. J Clin Oncol 33 (26): 2893-900, 2015.
-
Madenci AL, Dieffenbach BV, Liu Q, et al.: Late-onset anorectal disease and psychosocial impact in survivors of childhood cancer: A report from the Childhood Cancer Survivor Study. Cancer 125 (21): 3873-3881, 2019.
-
Heyn R, Raney RB, Hays DM, et al.: Late effects of therapy in patients with paratesticular rhabdomyosarcoma. Intergroup Rhabdomyosarcoma Study Committee. J Clin Oncol 10 (4): 614-23, 1992.
-
Hughes LL, Baruzzi MJ, Ribeiro RC, et al.: Paratesticular rhabdomyosarcoma: delayed effects of multimodality therapy and implications for current management. Cancer 73 (2): 476-82, 1994.
-
Raney B, Heyn R, Hays DM, et al.: Sequelae of treatment in 109 patients followed for 5 to 15 years after diagnosis of sarcoma of the bladder and prostate. A report from the Intergroup Rhabdomyosarcoma Study Committee. Cancer 71 (7): 2387-94, 1993.
-
Asdahl PH, Oeffinger KC, Albieri V, et al.: Esophageal disease among childhood cancer survivors-A report from the Childhood Cancer Survivors Study. Pediatr Blood Cancer 68 (8): e29043, 2021.
-
Mulder RL, van Dalen EC, Van den Hof M, et al.: Hepatic late adverse effects after antineoplastic treatment for childhood cancer. Cochrane Database Syst Rev (7): CD008205, 2011.
-
Castellino S, Muir A, Shah A, et al.: Hepato-biliary late effects in survivors of childhood and adolescent cancer: a report from the Children's Oncology Group. Pediatr Blood Cancer 54 (5): 663-9, 2010.
-
Mulder RL, Kremer LC, Koot BG, et al.: Surveillance of hepatic late adverse effects in a large cohort of long-term survivors of childhood cancer: prevalence and risk factors. Eur J Cancer 49 (1): 185-93, 2013.
-
Green DM, Wang M, Krasin MJ, et al.: Serum Alanine Aminotransferase Elevations in Survivors of Childhood Cancer: A Report From the St. Jude Lifetime Cohort Study. Hepatology 69 (1): 94-106, 2019.
-
Castellino SM, Hudson MM: Late gastrointestinal and hepatic effects. In: Schwartz CL, Hobbie WL, Constine LS, et al., eds.: Survivors of Childhood and Adolescent Cancer: A Multidisciplinary Approach. 3rd ed. Springer International Publishing, 2015, pp 229-52.
-
Mahmoud H, Schell M, Pui CH: Cholelithiasis after treatment for childhood cancer. Cancer 67 (5): 1439-42, 1991.
-
Safford SD, Safford KM, Martin P, et al.: Management of cholelithiasis in pediatric patients who undergo bone marrow transplantation. J Pediatr Surg 36 (1): 86-90, 2001.
-
Dieffenbach BV, Li N, Madenci AL, et al.: Incidence of and risk factors for late cholecystectomy in survivors of childhood cancer: A report from the Childhood Cancer Survivor Study. Eur J Cancer 133: 4-13, 2020.
-
Sudour H, Mainard L, Baumann C, et al.: Focal nodular hyperplasia of the liver following hematopoietic SCT. Bone Marrow Transplant 43 (2): 127-32, 2009.
-
Lee MH, Yoo SY, Kim JH, et al.: Hypervascular hepatic nodules in childhood cancer survivors: clinical and imaging features. Clin Imaging 36 (4): 301-7, 2012 Jul-Aug.
-
Wanless IR: Micronodular transformation (nodular regenerative hyperplasia) of the liver: a report of 64 cases among 2,500 autopsies and a new classification of benign hepatocellular nodules. Hepatology 11 (5): 787-97, 1990.
-
Brisse H, Servois V, Bouche B, et al.: Hepatic regenerating nodules: a mimic of recurrent cancer in children. Pediatr Radiol 30 (6): 386-93, 2000.
-
Chu WC, Roebuck DJ: Nodular regenerative hyperplasia of the liver simulating metastases following treatment for bilateral Wilms tumor. Med Pediatr Oncol 41 (1): 85-7, 2003.
-
Halonen P, Mattila J, Ruuska T, et al.: Liver histology after current intensified therapy for childhood acute lymphoblastic leukemia: microvesicular fatty change and siderosis are the main findings. Med Pediatr Oncol 40 (3): 148-54, 2003.
-
Tomita Y, Ishiguro H, Yasuda Y, et al.: High incidence of fatty liver and insulin resistance in long-term adult survivors of childhood SCT. Bone Marrow Transplant 46 (3): 416-25, 2011.
-
Ruccione KS, Wood JC, Sposto R, et al.: Characterization of transfusion-derived iron deposition in childhood cancer survivors. Cancer Epidemiol Biomarkers Prev 23 (9): 1913-9, 2014.
-
Vag T, Kentouche K, Krumbein I, et al.: Noninvasive measurement of liver iron concentration at MRI in children with acute leukemia: initial results. Pediatr Radiol 41 (8): 980-4, 2011.
-
Schempp A, Lee J, Kearney S, et al.: Iron Overload in Survivors of Childhood Cancer. J Pediatr Hematol Oncol 38 (1): 27-31, 2016.
-
Broxson EH, Dole M, Wong R, et al.: Portal hypertension develops in a subset of children with standard risk acute lymphoblastic leukemia treated with oral 6-thioguanine during maintenance therapy. Pediatr Blood Cancer 44 (3): 226-31, 2005.
-
Green DM, Norkool P, Breslow NE, et al.: Severe hepatic toxicity after treatment with vincristine and dactinomycin using single-dose or divided-dose schedules: a report from the National Wilms' Tumor Study. J Clin Oncol 8 (9): 1525-30, 1990.
-
Sulis ML, Bessmertny O, Granowetter L, et al.: Veno-occlusive disease in pediatric patients receiving actinomycin D and vincristine only for the treatment of rhabdomyosarcoma. J Pediatr Hematol Oncol 26 (12): 843-6, 2004.
-
McDonald GB: Hepatobiliary complications of hematopoietic cell transplantation, 40 years on. Hepatology 51 (4): 1450-60, 2010.
-
Dawson LA, Ten Haken RK: Partial volume tolerance of the liver to radiation. Semin Radiat Oncol 15 (4): 279-83, 2005.
-
Milano MT, Constine LS, Okunieff P: Normal tissue tolerance dose metrics for radiation therapy of major organs. Semin Radiat Oncol 17 (2): 131-40, 2007.
-
Pan CC, Kavanagh BD, Dawson LA, et al.: Radiation-associated liver injury. Int J Radiat Oncol Biol Phys 76 (3 Suppl): S94-100, 2010.
-
Bhanot P, Cushing B, Philippart A, et al.: Hepatic irradiation and adriamycin cardiotoxicity. J Pediatr 95 (4): 561-3, 1979.
-
Flentje M, Weirich A, Pötter R, et al.: Hepatotoxicity in irradiated nephroblastoma patients during postoperative treatment according to SIOP9/GPOH. Radiother Oncol 31 (3): 222-8, 1994.
-
Kun LE, Camitta BM: Hepatopathy following irradiation and adriamycin. Cancer 42 (1): 81-4, 1978.
-
Tefft M: Radiation related toxicities in National Wilms' Tumor Study Number 1. Int J Radiat Oncol Biol Phys 2 (5-6): 455-63, 1977 May-Jun.
-
Hall MD, Howell RM, Jackson A, et al.: Liver Late Effects in Childhood Cancer Survivors Treated With Radiation Therapy: A PENTEC Comprehensive Review. Int J Radiat Oncol Biol Phys 119 (2): 575-587, 2024.
-
Levitsky J, Sorrell MF: Hepatic complications of hematopoietic cell transplantation. Curr Gastroenterol Rep 9 (1): 60-5, 2007.
-
Pidala J, Chai X, Kurland BF, et al.: Analysis of gastrointestinal and hepatic chronic graft-versus-host [corrected] disease manifestations on major outcomes: a chronic graft-versus-host [corrected] disease consortium study. Biol Blood Marrow Transplant 19 (5): 784-91, 2013.
-
Tomás JF, Pinilla I, García-Buey ML, et al.: Long-term liver dysfunction after allogeneic bone marrow transplantation: clinical features and course in 61 patients. Bone Marrow Transplant 26 (6): 649-55, 2000.
-
Aricò M, Maggiore G, Silini E, et al.: Hepatitis C virus infection in children treated for acute lymphoblastic leukemia. Blood 84 (9): 2919-22, 1994.
-
Castellino S, Lensing S, Riely C, et al.: The epidemiology of chronic hepatitis C infection in survivors of childhood cancer: an update of the St Jude Children's Research Hospital hepatitis C seropositive cohort. Blood 103 (7): 2460-6, 2004.
-
Cesaro S, Petris MG, Rossetti F, et al.: Chronic hepatitis C virus infection after treatment for pediatric malignancy. Blood 90 (3): 1315-20, 1997.
-
Fink FM, Höcker-Schulz S, Mor W, et al.: Association of hepatitis C virus infection with chronic liver disease in paediatric cancer patients. Eur J Pediatr 152 (6): 490-2, 1993.
-
Locasciulli A, Testa M, Pontisso P, et al.: Hepatitis C virus genotypes and liver disease in patients undergoing allogeneic bone marrow transplantation. Bone Marrow Transplant 19 (3): 237-40, 1997.
-
Locasciulli A, Testa M, Pontisso P, et al.: Prevalence and natural history of hepatitis C infection in patients cured of childhood leukemia. Blood 90 (11): 4628-33, 1997.
-
Paul IM, Sanders J, Ruggiero F, et al.: Chronic hepatitis C virus infections in leukemia survivors: prevalence, viral load, and severity of liver disease. Blood 93 (11): 3672-7, 1999.
-
Lansdale M, Castellino S, Marina N, et al.: Knowledge of hepatitis C virus screening in long-term pediatric cancer survivors: a report from the Childhood Cancer Survivor Study. Cancer 116 (4): 974-82, 2010.
-
van Waas M, Neggers SJ, Raat H, et al.: Abdominal radiotherapy: a major determinant of metabolic syndrome in nephroblastoma and neuroblastoma survivors. PLoS One 7 (12): e52237, 2012.
-
Neville KA, Cohn RJ, Steinbeck KS, et al.: Hyperinsulinemia, impaired glucose tolerance, and diabetes mellitus in survivors of childhood cancer: prevalence and risk factors. J Clin Endocrinol Metab 91 (11): 4401-7, 2006.
-
Baker KS, Ness KK, Steinberger J, et al.: Diabetes, hypertension, and cardiovascular events in survivors of hematopoietic cell transplantation: a report from the bone marrow transplantation survivor study. Blood 109 (4): 1765-72, 2007.
-
Dixon SB, Wang F, Lu L, et al.: Prediabetes and Associated Risk of Cardiovascular Events and Chronic Kidney Disease Among Adult Survivors of Childhood Cancer in the St Jude Lifetime Cohort. J Clin Oncol 42 (9): 1031-1043, 2024.
-
Friedman DN, Moskowitz CS, Hilden P, et al.: Radiation Dose and Volume to the Pancreas and Subsequent Risk of Diabetes Mellitus: A Report from the Childhood Cancer Survivor Study. J Natl Cancer Inst 112 (5): 525-532, 2020.
-
Williams HE, Howell CR, Chemaitilly W, et al.: Diabetes mellitus among adult survivors of childhood acute lymphoblastic leukemia: A report from the St. Jude Lifetime Cohort Study. Cancer 126 (4): 870-878, 2020.
-
de Vathaire F, El-Fayech C, Ben Ayed FF, et al.: Radiation dose to the pancreas and risk of diabetes mellitus in childhood cancer survivors: a retrospective cohort study. Lancet Oncol 13 (10): 1002-10, 2012.
-
van Nimwegen FA, Schaapveld M, Janus CP, et al.: Risk of diabetes mellitus in long-term survivors of Hodgkin lymphoma. J Clin Oncol 32 (29): 3257-63, 2014.
Late Effects of the Endocrine System
Endocrine dysfunction is common among survivors of childhood cancer, especially in those who were treated with surgery or radiation therapy that involved hormone-producing organs and those who received alkylating agent chemotherapy.
Figure 6. Prevalence of endocrine disorders at the last follow-up visit, by sex. Brignardello E, Felicetti F, Castiglione A, et al.: Endocrine health conditions in adult survivors of childhood cancer: the need for specialized adult-focused follow-up clinics. European Journal of Endocrinology 168 (3): 465-472, 2013. Copyright © 2013, European Society of Endocrinology.
The prevalence of specific endocrine disorders is affected by the following:[1,2,3,4]
-
Patient factors (e.g., age at treatment and sex).
-
Tumor location (e.g., suprasellar and nonsuprasellar).[5]
-
Treatment factors (e.g., radiation dose and treatment volume).
-
Time from radiation exposure (typically increases with longer time from radiation exposure [see Figure 6]).[1]
Endocrinologic late effects can be broadly categorized as those resulting from hypothalamic-pituitary injury or from peripheral glandular compromise.[1,2,3,4] The former are most common after treatment for central nervous system (CNS) tumors. The prevalence of these late effects was 24.8% in a nationwide cohort study of 718 survivors who lived longer than 2 years and all hypothalamic-pituitary axes were affected.[3]
The following sections summarize research that characterizes the clinical features of survivors at risk of endocrine dysfunction that impacts pituitary, thyroid, adrenal, and gonadal function.
Thyroid Gland
- Thyroid dysfunction is a common delayed effect after radiation therapy exposures to fields that include the thyroid gland. This type of radiation therapy is used in treating Hodgkin lymphoma, brain tumors, head and neck sarcomas, and acute lymphoblastic leukemia (ALL).
- There is considerable evidence linking radiation exposure to thyroid abnormalities. However, the prevalence of specific conditions varies widely because studies are limited by cohort selection and participation bias, heterogeneity in radiation treatment approach, time since radiation exposure, and method of ascertainment (e.g., self-report vs. clinical or diagnostic imaging assessment).
- Thyroid abnormalities observed in excess in childhood cancer survivors include the following:
- Primary hypothyroidism.
- Hyperthyroidism.
- Goiter.
- Nodules.
Hypothyroidism
Risk factors
Thyroid radiation therapy. An increased risk of hypothyroidism has been reported among childhood cancer survivors treated with head and neck radiation exposing the thyroid gland, especially among survivors of Hodgkin lymphoma.[1,2,3,4] Among survivors of head and neck tumors treated with radiation therapy potentially exposing the hypothalamic-pituitary region and the thyroid gland, hypothyroidism may result from thyrotropin-releasing hormone (TRH) and/or thyroid-stimulating hormone (TSH) deficiency (central hypothyroidism), thyroid gland dysfunction (primary hypothyroidism), or a combination of central and primary causes.
Iodine I 131-metaiodobenzylguanidine (131I-MIBG).
-
Therapeutic 131I-MIBG. Thyroid disorders, including primary hypothyroidism, have been reported in children with neuroblastoma who were treated with 131I-MIBG, despite thyroid protection with potassium iodide, perchlorate, or the combination of potassium iodide, thyroxine (T4), and a thiamazole. However, these reports feature small numbers of patients who have other treatment-related risk factors for thyroid disorders, which limits the ability to identify an independent effect of 131I-MIBG.[6] Thyroid disorders were diagnosed in 81% of survivors 15 years after treatment with 131I-MIBG.[7]
-
Diagnostic 131I-MIBG. Late-onset thyroid abnormalities have not been reported among childhood cancer survivors who had either 131I-MIBG or 123I-MIBG to monitor disease status.[8,9]
- A retrospective cohort study (n = 48) assessed the prevalence of thyroid dysfunction in survivors of a neuroblastic tumor who received 123I-MIBG for diagnostic purposes. All patients received thyroid prophylaxis (potassium iodide or combination of potassium iodide, thiamazole, and T4) during exposure to 123I-MIBG. After a median follow up of 6.6 years, thyroid function was normal in 46 of the 48 survivors. Two survivors had mild thyroid dysfunction.[10]
Thyroidectomy. As observed in the general, noncancer population, partial or obviously subtotal surgical resection of the thyroid is a risk factor for subsequent hypothyroidism.[11,12,13]
Evidence (prevalence of and risk factors for hypothyroidism):
- The German Group of Paediatric Radiation Oncology reported on 1,086 patients treated at 62 centers, including 404 patients (median age, 10.9 years) who received radiation therapy to the thyroid and/or pituitary gland.[14] Follow-up information was available for 264 patients (60.9%; median follow-up, 40 months), with 60 patients (22.7%) showing pathological values.
- Compared with patients treated with prophylactic cranial irradiation (median dose, 12 Gy), the hazard ratio (HR) for the development of pathological thyroid blood values was 3.072 (P = .002) for patients treated with radiation doses of 15 Gy to 25 Gy to the thyroid gland.
- The HR was 3.769 (P = .009) for patients treated with more than 25 Gy of radiation to the thyroid gland. The HR was 5.674 (P < .001) for patients treated with craniospinal irradiation (CSI).
- The cumulative incidence of thyroid hormone substitution therapy did not differ between defined subgroups.
- The Childhood Cancer Survivor Study (CCSS) investigated the prevalence of self-reported hypothyroidism assessed through serial questionnaires in 12,015 survivors. A total of 1,193 cases of hypothyroidism were observed, 777 (65%) of which occurred 5 or more years after a cancer diagnosis.[15]
- The prevalence at 5 years after a cancer diagnosis and the incidence through 30 years after a cancer diagnosis was the highest in 5-year survivors of Hodgkin lymphoma (32.3%) and CNS tumors (17.7%).
- The incidence was significantly associated with radiation dose to the thyroid and pituitary. The combined effects of thyroid and hypothalamic-pituitary doses appear to be less than additive when pituitary doses are greater than 16 Gy.
- Radiation-related risks were higher in males than females and inversely associated with age at exposure and time since exposure but remained elevated more than 25 years after exposure.
- Certain types of chemotherapy were significantly associated with the risk: bleomycin (rate ratio, 3.4), and the alkylating agents lomustine (rate ratio, 3.0) and cyclophosphamide (rate ratio, 1.3). The strongest chemotherapy-associated risk occurred among survivors of CNS cancer. A significant dose response for lomustine was observed (P < .01).
- In the CCSS, a cohort of childhood Hodgkin lymphoma survivors treated between 1970 and 1986 were evaluated for thyroid disease by use of a self-report questionnaire.[16]
- Among 1,791 survivors who were followed for a median of 14 years, 34% reported that they had been diagnosed with at least one thyroid abnormality.
- For hypothyroidism, there was a clear dose response, with a 20-year risk of:
- 20% for those who received less than 35 Gy of radiation to the thyroid gland.
- 30% for those who received 35 Gy to 44.9 Gy of radiation to the thyroid gland.
- 50% for those who received more than 45 Gy of radiation to the thyroid gland.
- Compared with a sibling control group, the relative risk (RR) was 17.1 for hypothyroidism.
- Elapsed time since diagnosis was a risk factor for hypothyroidism, with the risk increasing in the first 3 to 5 years postdiagnosis.
- Females were at increased risk for hypothyroidism.
- A follow-up study from the CCSS compared self-reported data from 14,290 survivors with data from 4,031 sibling controls.[2]
- The RR was 3.8 for hypothyroidism and remained significantly higher in survivors when compared with controls, even in the absence of radiation therapy to the thyroid or pituitary.
- These results indicate the need for continued and individualized long-term monitoring strategies in childhood cancer survivors.
- Continuous improvements in the precision of radiation therapy delivery carry the promise to decrease the radiation therapy dose received by the thyroid in a subset of patients. This was demonstrated in a study of 189 children and young adults (aged <26 years) with brain tumors treated with proton radiation therapy.[17]
- At a median follow-up of 4.4 years, the cumulative incidence of primary hypothyroidism was 3% after CSI and 1.6% overall, which is substantially lower than previous reports of 56% to 65% incidence after CSI with photons.
- Among 118 survivors of medulloblastoma (median follow-up, 5.6 years), the estimated cumulative incidence of hypothyroidism was 35.8% (95% confidence interval [CI], 26.7%–47.1%) by 5 years after radiation therapy.[18]
- The median time from the end of radiation therapy to the development of hypothyroidism was 2.1 years (range, 0.1–7.1 years) after proton radiation therapy and 2.9 years (range, 0.8–7.2 years) after photon radiation therapy.
- The rate of hypothyroidism (HR, 2.36; 95% CI, 1.11–5.02) was higher among patients treated with photon radiation therapy compared with those treated with proton radiation therapy.
- Differences in hypothyroidism risk by radiation modality were largely attributed to higher rates of primary hypothyroidism after photon radiation therapy (HR, 4.61; 95% CI, 1.20–17.66).
- St. Jude Life (SJLIFE) cohort study investigators evaluated the prevalence of and risk factors for primary hypothyroidism and its associations with chronic health conditions and quality of life among 2,965 childhood cancer survivors (median age, 30.9 years; median time postdiagnosis, 22.3 years).[13]
- Primary hypothyroidism was prevalent in 14.7% of survivors. It was more common in females (odds ratio [OR], 1.06; 95% CI, 1.03–1.08) and survivors diagnosed older than 15 years (vs. age <5 years). It was less common in non-White survivors (OR, 0.96; 95% CI, 0.93–0.99).
- Radiation therapy potentially exposing the thyroid gland was associated with dose-related risk of hypothyroidism.
- Significant associations with primary hypothyroidism included frailty (OR, 1.54; 95% CI, 1.05–2.26), dyslipidemia (OR, 1.52; 95% CI, 1.14–2.04), and impaired physical quality of life (OR, 1.66; 95% CI, 1.12–2.48).
- The Pediatric Normal Tissue Effects in the Clinic (PENTEC) research consortium performed a systematic review of radiation-associated primary hypothyroidism in children younger than 21 years between 1970 and 2017.[19]
- At mean doses of 10 Gy, 20 Gy, and 30 Gy, predicted rates of uncompensated (clinical) hypothyroidism were 4%, 7%, and 13%, respectively. The compensated (subclinical) hypothyroidism rates were 12%, 25% and 44%, respectively.
- Female sex (RR, 1.7; P < .0001) and age younger than 15 years at the time of radiation therapy (RR, 1.3; P < .005) were associated with higher risks of hypothyroidism.
- After a mean thyroid dose of 20 Gy, predicted rates of hypothyroidism were 13% for males younger than 14 years and more than 29% for females older than 15 years at the time of radiation therapy.
Table 8. Risks of Hypothyroidism (Compensated or Uncompensated) After Radiation Therapy for Pediatric Malignancies, Grouped by Mean Thyroid Dose, Age, and SexaMean Thyroid Dose | Risk of Hypothyroidismb |
---|
Age <14 yc | Age >15 yc |
---|
Female | Male | Female | Male |
---|
a Adapted from Milano et al.[19] |
b Any hypothyroidism (i.e., compensated or uncompensated). |
c Age 14 to 15 y was used as a cutoff because the two studies that analyzed age used different cut-points. Presumably, the risks of hypothyroidism in patients irradiated at ages 14 to 15 y would be intermediate to those shown for ages <14 y and >15 y. |
10 Gy | 10% | 6% | 14% | 8% |
20 Gy | 22% | 13% | 29% | 17% |
30 Gy | 39% | 23% | 53% | 31% |
40 Gy | 59% | 35% | 79% | 47% |
- The PENTEC research consortium performed a systematic review of radiation-associated hypothyroidism.[20]
- Among seven cohorts (250 patients), the dose associated with a 50% risk (D50) of hypothyroidism was 39 Gy (95% CI, 34.1–53.2).
- The risk was 20% for children who received a mean dose of 22 Gy in 2-Gy fractions.
Clinical presentation
- Most children treated with radiation therapy who develop hypothyroidism do so within the first 2 to 5 years posttreatment, but new cases can occur later.
- Reports of thyroid dysfunction differ depending on the dose of radiation, the length of follow-up, and the biochemical criteria used to make the diagnosis.[21]
- The most frequently reported abnormalities include the following:
- Elevated TSH.
- Depressed T4.
- Elevated TSH and depressed T4.
- Central hypothyroidism results from radiation exposure of the hypothalamic pituitary axis; there is typically a low free T4 in conjunction with low TSH.
- Compensated hypothyroidism includes an elevated TSH with a normal T4 and is asymptomatic. The natural history is unclear, but most endocrinologists support treatment.
- Uncompensated hypothyroidism includes both an elevated TSH and a depressed T4.
- Thyroid hormone replacement is beneficial for correction of the metabolic abnormality, and has clinical benefits for cardiovascular, gastrointestinal, and neurocognitive function.
Hyperthyroidism
While less common than hypothyroidism, childhood cancer survivors also experience an increased risk of hyperthyroidism.[2,16,22,23]
Evidence (prevalence of and risk factors for hyperthyroidism):
- CCSS investigators evaluated the prevalence of thyroid disease among 1,791 childhood Hodgkin lymphoma survivors treated between 1970 and 1986 and followed for a median of 14 years.[16]
- Hyperthyroidism was reported by 5% of survivors, which was eightfold greater than the incidence reported by the controls.
- Radiation dose of 35 Gy or more to the thyroid was the only risk factor identified for hyperthyroidism.
- Another CCSS study evaluated the risk of hyperthyroidism in relation to incidental therapeutic radiation dose to the thyroid and pituitary glands.[22]
- Hyperthyroidism was self-reported by 179 survivors, with 148 cases diagnosed 5 or more years after cancer diagnosis.
- The cumulative proportion of survivors with hyperthyroidism by 30 years after cancer diagnosis was 2.5% (95% CI, 2.0%–2.9%).
- Thyroid radiation increased the risk of hyperthyroidism with evidence of a linear thyroid radiation dose-response over the dose range of 0 Gy to 63 Gy.
- Radiation to the pituitary gland and chemotherapy were not significantly associated with hyperthyroidism.
- Radiation-associated risk of hyperthyroidism remained elevated longer than 25 years after exposure.
Thyroid nodules
The clinical manifestation of thyroid neoplasia among childhood cancer survivors ranges from asymptomatic, small, solitary nodules to large, intrathoracic goiters that compress adjacent structures.
The following factors are linked to an increased risk of thyroid nodule development:
-
Radiation dose, time from diagnosis, and female sex.
- Any radiation field that includes the thyroid is associated with an excess risk of thyroid neoplasms, which may be benign (usually adenomas) or malignant (most often differentiated papillary carcinoma).[2,16,24,25,26,27]
- A CCSS study that included survivors of Hodgkin lymphoma identified time from diagnosis, female sex, and radiation dose of 25 Gy or higher as significant risk factors for thyroid nodule development.[16]
- Based on a cohort of 3,254 2-year childhood cancer survivors treated before 1986 and monitored for 25 years, the risk of thyroid adenoma increased with the size of the radiation dose to the thyroid during childhood cancer treatment and plateaued at doses exceeding 10 Gy.[25]
- CCSS investigators performed a nested case-control study to evaluate the magnitude of risk for thyroid cancer over the therapeutic radiation dose range of pediatric cancers. The risk of thyroid cancer increased with radiation doses up to 20 Gy to 29 Gy (OR, 9.8; 95% CI, 3.2–34.8), but declined at doses higher than 30 Gy, consistent with a cell-killing effect.[27]
-
Age at time of radiation therapy.
- Based on the same cohort of 3,254 2-year childhood cancer survivors, the risk of thyroid adenoma per unit of radiation dose to the thyroid was higher if radiation therapy had been delivered before age 5 years. The risk was also higher in individuals who were younger than 40 years at the time of the study.[25]
- Younger age at time of radiation therapy has also been linked to an excess risk of thyroid carcinoma.[24,25,26,27]
-
Exposure to 131I-MIBG.
- During childhood and adolescence, there is an increased risk of developing thyroid nodules, and potentially thyroid cancer, in patients exposed to 131I-MIBG.
- Clinicians caring for survivors treated with 131I-MIBG should be aware of the risks of developing thyroid dysfunction, thyroid nodules, and thyroid cancer.[28]
-
Chemotherapy.
- An increased risk of thyroid nodules and cancer has also been observed in association with chemotherapy, independent of radiation exposure.[2,24,25]
- In a pooled study of two cohorts of 16,757 survivors that included 187 patients with secondary thyroid cancer, treatments with alkylating agents, anthracyclines, or bleomycin were associated with a significantly increased risk of thyroid cancer in individuals not exposed to radiation therapy.[29]
- In the CCSS, the rate ratio of developing thyroid cancer was 2.5 (P < .01) in survivors not treated with thyroid radiation when compared with sibling controls.[2]
- Areas of active research include defining the precise role of exposure to chemotherapy and developing risk prediction models for thyroid cancer in childhood cancer survivors on the basis of demographic and treatment-related risk factors.[30]
Screening for thyroid cancer
- Several studies have demonstrated that ultrasonography is superior to clinical examination for detecting thyroid nodules and thyroid cancers, and they have characterized ultrasonographic features of nodules that are more likely to be malignant.[31,32,33]
- Primary screening for thyroid neoplasia (beyond physical examination with thyroid palpation) remains controversial because of the lack of data indicating a survival benefit and quality-of-life benefit associated with early detection and intervention.[34]
- Because these lesions tend to be indolent, are rarely life-threatening, and may clinically manifest many years after exposure to radiation, there are significant concerns regarding the costs and harms of overscreening.[35]
- Expert panels have refrained from specifically endorsing or discouraging the use of ultrasonography as a screening tool for thyroid cancer and this continues to be an active area of investigation.[36]
- Following a systematic assessment of the evidence, the International Guideline Harmonization Group concluded that initiation of surveillance and the type of surveillance modality (thyroid palpation vs. ultrasonography) should be determined by shared decision-making between the health care provider and survivor after carefully considering the benefits and harms. A decision aid to facilitate discussion accompanies their recommendations.[34]
For information about subsequent thyroid cancers, see the Subsequent Neoplasms section.
Posttransplant thyroid dysfunction
Survivors of pediatric hematopoietic stem cell transplant (HSCT) are at increased risk of thyroid dysfunction.[37,38]
- In a retrospective study of a single pediatric transplant unit, 244 pediatric patients were observed over a 20-year period. These patients had undergone HSCT for malignant or nonmalignant diseases between 1999 and 2018. Patients were assessed with a minimum of four thyroid function tests and one or more thyroid ultrasounds sequentially after HSCT.[38]
- Thyroid complications were detected in 19.7% of patients.
- The 15-year cumulative incidence of either autoimmune or nonautoimmune thyroid dysfunctions (34%) did not differ statistically between patients who received total-body irradiation (TBI)–based regimens and patients who received chemotherapy-based regimens (P = .23). The cumulative incidence after busulfan-based conditioning was similar to that of TBI (10-year cumulative incidence, 22.2% vs. 25.9%, respectively).
- The cumulative incidence of nonautoimmune hypothyroidism was statistically higher after busulfan-based conditioning (12.4%) than after other chemotherapy-only–based conditioning regimens (3.1%; P = .02, 5-year cumulative incidence).
- The overall incidence of thyroid nodules was low for the first 5 years after HSCT (1.9%) but increased steeply over time, with a 15-year cumulative incidence as high as 52.1%. Patients who received TBI-based conditioning regimens had a higher 15-year cumulative incidence of nodules (66.8%) than patients who received chemotherapy-only regimens (33.6%; P = .02).
- Age older than 10 years at transplant showed a protective effect (HR, 0.42).
- Sonographic follow-up showed a progressive statistically significant reduction in thyroid anteroposterior diameter among patients who received TBI-based conditioning regimens (P = .005) but not in those who received chemotherapy-only regimens.
- In a report from the Fred Hutchinson Cancer Research Center, the risk of thyroid dysfunction after HSCT was much lower (15%–16%) after fractionated TBI, as opposed to single-dose TBI (46%–48%).[37]
- The increased risk of thyroid dysfunction did not differ between children receiving a TBI-based or busulfan-based regimen (P = .48).
- Other high-dose therapies have not been studied.
TSH deficiency (central hypothyroidism) is discussed with late effects that affect the pituitary gland.
Table 9 summarizes thyroid late effects and the related health screenings.
Table 9. Thyroid Late Effectsa
Predisposing Therapy |
Endocrine/Metabolic Effects |
Health Screening |
131I-MIBG = Iodine I 131-metaiodobenzylguanidine; T4 = thyroxine; TSH = thyroid-stimulating hormone. |
a Adapted from theChildren's Oncology Group Long-Term Follow-Up Guidelines for Survivors of Childhood, Adolescent, and Young Adult Cancers. |
Radiation exposing thyroid gland; thyroidectomy |
Primary hypothyroidism |
TSH level |
Radiation exposing thyroid gland |
Hyperthyroidism |
Free T4 level |
TSH level |
Radiation exposing thyroid gland, including 131I-MIBG |
Thyroid nodules |
Thyroid examination |
Thyroid ultrasonography |
Hypothalamus-Pituitary Axis
Survivors of childhood cancer are at risk of developing a spectrum of neuroendocrine abnormalities, primarily because of the effect of radiation therapy on the hypothalamus.
- There are two categories of childhood cancer survivors who experience hypothalamus-pituitary dysfunction after radiation—survivors with a CNS tumor and survivors treated for a non-CNS tumor of the head or neck or leukemia and/or individuals with a history of an HSCT.[39]
- In addition, tumor development or surgical resection close to the hypothalamus and/or pituitary gland may induce direct anatomical damage to these structures and result in hypothalamic-pituitary dysfunction.
- Essentially all of the hypothalamic-pituitary axes are at risk.[40,41,42]
The quality of the literature regarding pituitary endocrinopathy among childhood cancer survivors is often limited by retrospective data collection, small sample size, cohort selection and participation bias, heterogeneity in treatment approach, time since treatment, and method of ascertainment. However, the evidence linking this outcome with radiation therapy, surgery, and tumor infiltration is compelling because affected individuals typically present with metabolic and developmental abnormalities early in follow-up.
The risk of hypothalamus-pituitary dysfunction increases with higher doses of radiation therapy. When the radiation therapy dose exceeds 30 Gy, there is a higher risk of developing hypothalamus-pituitary disorders, including adrenocorticotropic hormone (ACTH) deficiency, luteinizing hormone (LH)/follicle-stimulating hormone (FSH) deficiency, and TSH deficiency.[39]
Central diabetes insipidus
Central diabetes insipidus may herald the diagnosis of craniopharyngioma, suprasellar germ cell tumor, or Langerhans cell histiocytosis.[43,44,45]
- Diabetes insipidus may occur as an isolated pituitary deficiency at presentation of sellar/suprasellar tumors, although additional pituitary hormone deficiencies may develop with tumor progression.
- More commonly, diabetes insipidus occurs in the context of panhypopituitarism caused by the effects of the tumor on the hypothalamic-pituitary-adrenal (HPA) axis, or as a consequence of surgical procedures undertaken for local tumor control.
- Central diabetes insipidus is unlikely to occur as a late effect from surgical tumor resection past 2 years of follow-up.[46] Its emergence past that time should prompt assessment for tumor changes or other causes.
- Central diabetes insipidus has not been reported as a late effect of cranial irradiation in childhood cancer survivors.[3]
Anterior pituitary hormone deficiency
Deficiencies of anterior pituitary hormones and major hypothalamic regulatory factors are common late effects among survivors treated with cranial irradiation.[42]
Evidence (prevalence of anterior pituitary hormone deficiency):
- Young patients with brain tumors are particularly vulnerable to hypothalamic-pituitary dysfunction. One study included 718 childhood brain tumor survivors who were monitored for a median of 7.9 years.[47]
- No differences were observed in the prevalence of hypothalamic-pituitary dysfunction among infants (0–1 years), toddlers (≥1–3 years), or older age groups at diagnosis, despite the fact that radiation therapy was less frequently used in infants.
- Radiation exposure (OR, 16.44; 95% CI, 8.93–30.27), suprasellar tumor location (OR, 44.76; 95% CI, 19.00–105.49), and younger age (OR, 1.11; 95% CI, 1.05–1.18) were associated with hypothalamic dysfunction.
- Infant and toddler brain tumor survivors showed more weight gain (P < .0001) and smaller height standard deviation score (SDS) (P = .001) during follow-up than survivors diagnosed at an older age.
- Among non-irradiated survivors, those who were infants and toddlers at diagnosis were significantly more frequently diagnosed with TSH, ACTH, and antidiuretic hormone (ADH) deficiency, compared with survivors diagnosed at an older age, presumably caused by systemic therapies or the trauma of neurosurgery.
- A study targeting more refined data on the radiation doses associated with pituitary insufficiency analyzed 102 patients treated for tumors that included radiation therapy exposures (CNS, head and neck, hematologic malignancies) between 2008 and 2019. The median (interquartile range [IQR]) age at radiation therapy was 9.0 years (6.0–12.0 years), and the median follow-up was 5.7 years (3.5–9.1 years). Most patients received focal brain radiation therapy (36.3%) or TBI (32.4%). The most frequent diagnoses were ALL (25.5%) and medulloblastoma (17.6%).[48]
- Most patients developed pituitary insufficiency (64 patients; 62.7%). Forty-one percent of patients had one hormone deficiency and 38% had two hormone deficiencies.
- Growth hormone deficiency (58 patients; 56.9%) and TSH deficiency (32 patients; 31.4%) were most common.
- Patients who developed pituitary insufficiency had received a higher maximum pituitary dose (median [IQR], 44.0 Gy [20.4–54.0] vs. 18.2 Gy [14.4–52.6]; P = .008). Doses of 40 to 49 Gy or higher than 50 Gy led to a higher cumulative incidence rate than doses lower than 20 Gy (HR, 4.07; P < .001 and HR, 3.04; P < .001, respectively).
- However, even at lower dose bands, levels of pituitary insufficiency were significant. The 5-year cumulative incidence was higher than 30% for growth hormone deficiency with doses lower than 20 Gy and for TSH deficiency with doses of 20 to 29 Gy.
- In a single-institution study, 1,713 adult survivors of childhood cancers and brain tumors (median age, 32 years) were monitored for a median follow-up of 25 years.[41]
- The prevalence of hypothalamic-pituitary axis disorders was 56.4% in individuals exposed to cranial radiation therapy at doses of 18 Gy or higher.
- A study of 3,141 childhood cancer survivors who were systematically evaluated for hypothalamic-pituitary disorders at a median follow-up of 24.1 years reported the following:[49]
- For patients who were treated with hypothalamic-pituitary radiation therapy (n = 1,089), the following was observed:
- The estimated prevalence of growth hormone deficiency was 40.2%.
- The estimated prevalence of TSH deficiency was 11.1%.
- The estimated prevalence of LH/FSH deficiency was 10.6%.
- The estimated prevalence of ACTH deficiency was 3.2%.
- The estimated prevalence of central precocious puberty was 0.9%.
- For patients who did not receive hypothalamic-pituitary radiation therapy, the estimated prevalence of growth hormone deficiency was 6.2% and less than 1% for the other hypothalamic-pituitary disorders.
- Clinical factors independently associated with hypothalamic-pituitary disorders included the following:
- Hypothalamic-pituitary radiation therapy (even at relatively low doses, except for ACTH deficiency which was not observed until >30 Gy).
- Alkylating agents (growth hormone deficiency, LH/FSH deficiency).
- Intrathecal chemotherapy (growth hormone deficiency).
- Hydrocephalus with shunt placement (growth hormone deficiency, LH/FSH deficiency).
- Seizures (TSH deficiency, ACTH deficiency).
- Stroke (growth hormone deficiency, TSH deficiency, LH/FSH deficiency, ACTH deficiency).
- Adverse outcomes associated with hypothalamic-pituitary disorders included the following:
- Short stature (growth hormone deficiency, TSH deficiency).
- Severe bone mineral deficit (growth hormone deficiency, LH/FSH deficiency).
- Obesity (LH/FSH deficiency).
- Frailty (growth hormone deficiency).
- Impaired physical health-related quality of life (TSH deficiency).
- Sexual dysfunction (LH/FSH deficiency).
- Impaired memory and processing speed (growth hormone deficiency, TSH deficiency).
The International Late Effects of Childhood Cancer Guideline Harmonization Group (IGHG) consisting of 42 interdisciplinary international experts performed a systematic literature search on hypothalamic-pituitary axis surveillance in childhood cancer survivors.[39]
- They noted that hypothalamic-pituitary axis radiation exposure increased the risk for hypothalamic-pituitary dysfunction, with a dose dependent relationship. Doses of radiation therapy higher than 18 Gy are associated with a higher risk for growth hormone deficiency and central precocious puberty.
- Deficiencies in ACTH, TSH, and LH/FSH generally occur after doses higher than 30 Gy.
- The panel noted that childhood cancer survivors who have had local tissue injury to the hypothalamic-pituitary region are at risk of developing hypothalamic-pituitary dysfunction from the time of diagnosis or surgery for a brain tumor located near or within the hypothalamic-pituitary region or from occurrence of hydrocephalus (specifically at risk for growth hormone deficiency and central precocious puberty).
- Individuals exposed to radiation are at risk of developing hypothalamic-pituitary dysfunction one year from completion of radiation therapy.
- Childhood cancer survivors remain particularly at risk of growth hormone deficiency for at least 15 years after cancer diagnosis or treatment exposure. The risk beyond 15 years depends on individual patient factors. For central precocious puberty, patients remain at risk until age 8 years in girls and age 9 years for boys.
The six anterior pituitary hormones and their major hypothalamic regulatory factors are outlined in Table 10.
Table 10. Anterior Pituitary Hormones and Major Hypothalamic Regulatory Factors
Pituitary Hormone |
Hypothalamic Factor |
Hypothalamic Regulation of the Pituitary Hormone |
(–) = inhibitory; (+) = stimulatory. |
Growth hormone (GH) |
GH-releasing hormone |
+ |
Somatostatin |
– |
Prolactin |
Dopamine |
– |
Luteinizing hormone (LH) |
Gonadotropin-releasing hormone |
+ |
Follicle-stimulating hormone (FSH) |
Gonadotropin-releasing hormone |
+ |
Thyroid-stimulating hormone (TSH) |
Thyroid-releasing hormone |
+ |
Somatostatin |
– |
Adrenocorticotropic hormone (ACTH) |
Corticotropin-releasing hormone |
+ |
Vasopressin |
+ |
Growth hormone deficiency
Growth hormone deficiency is the most common and often the first anterior pituitary deficit to occur after cranial radiation therapy. In childhood survivors of CNS tumors, the overall prevalence of growth hormone deficiency is 12.5%.[3]
- The risk increases with radiation dose and time since treatment.
- Growth hormone deficiency can result from relatively low doses of cranial radiation.[50]
- Growth hormone deficiency can develop after 18 Gy to 24 Gy of cranial radiation, which is used to treat patients with ALL and lymphoma. The higher the radiation dose, the earlier that growth hormone deficiency will occur after treatment.[51]
- Approximately 60% to 80% of pediatric patients with brain tumors who received radiation therapy doses higher than 30 Gy will have impaired serum growth hormone response to provocative stimulation, usually within 5 years of treatment.[52,53]
Evidence (radiation-dose response relationship of growth hormone deficiency in childhood brain tumor survivors):
- In a report of 156 patients with medulloblastoma who were treated from 2003 to 2013 in the SJMB03 study, the following was observed:[54]
- The 5-year cumulative incidence of growth hormone deficiency was 68.9%, and the cumulative incidence of growth hormone replacement was 48.1%.
- Growth hormone deficiency was associated with increased hypothalamus dose (HR, 1.035; P = .0055) in average-risk patients and in high-risk patients who had a cerebrospinal fluid shunt (HR, 2.532; P = .0049).
- A study of conformal radiation therapy (CRT) in children with CNS tumors indicates that growth hormone insufficiency can usually be demonstrated within 12 months of radiation therapy, depending on hypothalamic dose-volume effects.[55]
- In a report featuring data from 118 patients with localized brain tumors who were treated with radiation therapy, peak growth hormone was modeled as an exponential function of time after CRT and mean radiation dose to the hypothalamus.[53]
- The average patient was predicted to develop growth hormone deficiency with the following combinations of time after CRT and mean dose to the hypothalamus: 12 months and more than 60 Gy; 36 months and 25 Gy to 30 Gy; and 60 months and 15 Gy to 20 Gy.
- A cumulative dose of 16.1 Gy to the hypothalamus would be considered the mean radiation dose causing a 50% risk of growth hormone deficiency at 5 years (TD50/5) (see Figure 7).
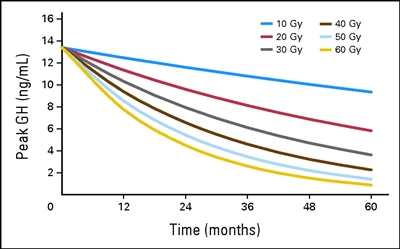
Figure 7. Peak growth hormone (GH) according to hypothalamic mean dose and time after start of radiation. According to equation 2, peak GH = exp{2.5947 + time × [0.0019 − (0.00079 × mean dose)]}. Thomas E. Merchant, Susan R. Rose, Christina Bosley, Shengjie Wu, Xiaoping Xiong, and Robert H. Lustig, Growth Hormone Secretion After Conformal Radiation Therapy in Pediatric Patients With Localized Brain Tumors, Journal of Clinical Oncology, volume 29, issue 36, pages 4776-4780. Reprinted with permission. © (2011) American Society of Clinical Oncology. All rights reserved.
- A PENTEC review reported on the risk of growth hormone deficiency for childhood cancer survivors treated with radiation therapy.[20]
- The normal tissue complication probability modeling for growth hormone deficiency (18 cohorts, 545 patients) found that the D50 was 24.9 Gy (95% CI, 20.9–28.0).
- The normal tissue complication probability model fit for whole-brain irradiation in children with a median age of older than 5 years indicated a 20% risk of growth hormone deficiency for patients who received a mean dose of 21 Gy in 2-Gy fractions to the hypothalamic-pituitary axis.
Evidence (risk of growth deficits in childhood cancer survivors):
- One study evaluated 127 patients with ALL treated with 24 Gy, 18 Gy, or no cranial radiation therapy (treatment era, 1980s–1990s).[56]
- The change in height, compared with population norms expressed as the SDS, was significant for all three groups. The dose response was -0.49 ± 0.14 for the group that did not receive radiation therapy, -0.65 ± 0.15 for the group that received 18 Gy of radiation therapy, and -1.38 ± 0.16 for the group that received 24 Gy of radiation therapy.
- Survivors of childhood ALL who are treated with chemotherapy alone are also at increased risk for adult short stature, although the risk is highest for those treated with cranial and craniospinal radiation therapy at a young age.[57] In a cross-sectional study, attained adult height was determined for 2,434 ALL survivors participating in the CCSS.
- All survivor treatment exposure groups (chemotherapy alone and chemotherapy with cranial or craniospinal radiation therapy) had decreased adult height and an increased risk of adult short stature (height SDS < -2), compared with siblings (P < .001).
- Compared with siblings, the risk of short stature for survivors treated with chemotherapy alone was elevated (OR, 3.4; 95% CI, 1.9–6.0).
- Among survivors, significant risk factors for short stature included diagnosis of ALL before puberty, higher-dose cranial radiation therapy (≥20 Gy vs. <20 Gy), any radiation therapy to the spine (which effects vertebral bodies), and female sex.
- The impact of chemotherapy alone on growth in 67 survivors treated with contemporary regimens for ALL was statistically significant at -0.59 SD. The loss of growth potential did not correlate with growth hormone status in this study, further highlighting the participation of other factors in the growth impairments observed in this population.[58]
- In a longitudinal study of 372 survivors of ALL who were treated on a single-institution chemotherapy-only trial, the following were observed:[59]
- Height z-scores declined during treatment and improved after therapy.
- Younger age at diagnosis (2 to <10 years), low-risk ALL status, white blood cell count below 50 × 109 /L at diagnosis, or CNS-negative status were associated with significant improvements in z-scores for height during the off-therapy period compared with those older at diagnosis (age ≥10 years), those with standard-risk/high-risk ALL status, those with a white blood cell count of 50 × 109 /L or higher, or CNS-positive status.
- The loss in height potential in older patients was attributed to attenuation of the growth spurt during treatment without improvement after therapy and to chemotherapy intensity in patients with standard- or high-risk disease features.
- A French CCSS study evaluated risk factors for short adult height and growth hormone deficiency in 2,965 childhood cancer survivors using clinical and treatment data available in medical records. Patients treated with growth hormones (6.4%) were excluded.[60]
- Independent risk factors for short adult height included being young at the time of cancer treatment (RR, 0.9 per year of age), having a short height at diagnosis (≤2 SDS: RR, 6.7), and having received treatment with pituitary radiation (5–20 Gy: RR, 4.2; 20–40 Gy: RR, 10.2; ≥40 Gy: RR, 19.5), busulfan (RR, 4.5), or more than 300 mg/m2 of lomustine (300–600 mg/m2: RR, 4.2 and ≥600 mg/m2: RR, 9.1).
- Children who were treated with 15 Gy or higher of radiation to 90% or more volume of seven or more vertebrae without pituitary irradiation had an increased risk of short adult stature (RR, 4.6). This risk increased by 1.3-fold to 2.4-fold if they also received pituitary radiation therapy.
- A St. Jude Children's Research Hospital investigation evaluated long-term (median follow-up, 10.2 years) height outcomes among 212 pediatric patients with CNS embryonal tumors who were treated with CSI at 23.4 Gy (n = 147) or 36 Gy or higher (n = 65).[61]
- Compared with U.S. standards, mean final height z-scores at age 18 years were -1.3 for female and -1.5 for male survivors.
- Factors associated with risk of height impairment included younger age at the time of CSI, higher CSI dose, and female sex.
- Factors associated with higher growth rates before the age of 15 years included older age at CSI, male sex, CSI dose lower than 36 Gy, replacement therapy for growth hormone and central adrenal insufficiency, and White race.
Growth after hematopoietic stem cell transplant (HSCT)
- Children who undergo HSCT with TBI have a significant risk of both growth hormone deficiency and the direct effects of radiation on skeletal development.[62,63,64]
- The risk of growth hormone deficiency is increased with single-dose TBI as opposed to fractionated TBI, pretransplant cranial irradiation, female sex, and posttreatment complications such as graft-versus-host disease (GVHD).[62,63,64]
- Hyperfractionation of the TBI dose markedly reduces risk in patients who have not undergone cranial irradiation for CNS leukemia prophylaxis or therapy.[65]
- Regimens containing busulfan and cyclophosphamide appear to increase risk in some studies,[64,66] but not others.[67]
Evidence (growth hormone deficiency in childhood HSCT survivors):
- The late effects that occur after HSCT have been studied by the Late Effects Working Party of the European Group for Blood and Marrow Transplantation. Among 181 patients with aplastic anemia, leukemias, and lymphomas who underwent HSCT before puberty, the following results were observed:[68,69]
- An overall decrease in final height–SDS value was found, compared with height at transplant and genetic height. The mean loss of height is estimated to be approximately 1 height–SDS (6 cm), compared with the mean height at time of HSCT and mean genetic height.
- The type of transplant, GVHD, and growth hormone or steroid treatment did not influence final height.
- TBI (single-dose more than fractionated-dose radiation therapy), male sex, and young age at transplant were found to be major factors for long-term height loss.
- Most patients (140 of 181) reached adult height within the normal range of the general population.
- Growth hormone deficiency has been reported after lower doses with a single fraction of 10 Gy and fractionated doses of 12 to 18 Gy of TBI.[70]
Growth hormone replacement therapy
- Growth hormone replacement therapy provides the benefit of optimizing height outcomes among children who have not reached skeletal maturity.[42] The diagnosis requires specialized dynamic testing.[42,71]
- Treatment with recombinant growth hormone (rGH) replacement therapy is generally delayed until 12 months after successful completion of cancer or brain tumor treatments and after a multidisciplinary discussion involving the prescribing pediatric endocrinologist, the primary oncologist, and other providers selected by the patient or family.[72]
- Safety concerns pertaining to the use of rGH in childhood cancer survivors have primarily been related to the mitogenic potential of the growth hormone stimulating tumor growth in a population with an increased risk of second neoplasms.[73] Most studies that report these outcomes, however, are limited by selection bias and small sample size.
Evidence (subsequent neoplasm risk after growth hormone deficiency replacement therapy):
- One study evaluated 361 growth hormone–treated cancer survivors enrolled in the CCSS and compared risk of recurrence, risk of subsequent neoplasm, and risk of death among survivors who did and did not receive treatment with growth hormone.[74]
- The RR of disease recurrence was 0.83 (95% CI, 0.37–1.86) for growth hormone–treated survivors.
- Growth hormone–treated participants were diagnosed with 15 subsequent neoplasms, all solid tumors, for an overall RR of 3.21 (95% CI, 1.88–5.46). This was mainly due to a small excess number of subsequent neoplasms observed in survivors of acute leukemia.[74]
- With prolonged follow-up, the elevation of subsequent cancer risk resulting from growth hormone diminished.[75]
- Compared with survivors not treated with growth hormone, those who were treated had a twofold excess risk of developing a subsequent neoplasm (RR, 2.15; 95% CI, 1.33–3.47; P < .002). Meningiomas were the most commonly observed neoplasms (9 of 20 tumors).[74]
- A study from the CCSS reported on the risk of subsequent CNS neoplasms after a longer period of follow-up.[76]
- The adjusted rate ratio of meningioma and gliomas in growth hormone–treated survivors of CNS tumors was 1.0 (95% CI, 0.6–1.8; P = .94), when compared with CNS tumor survivors who were not treated with growth hormone. This finding indicates negligible differences between the two groups for this particular risk.
- A review of existing data suggests that treatment with growth hormone is not associated with an increased risk of CNS tumor progression or recurrence, or new or recurrent leukemia.[77]
In general, the data addressing subsequent malignancies among childhood cancer survivors treated with growth hormone therapy should be interpreted with caution given the small number of events.[42,55,72,73,74,78,79]
As outlined in a consensus statement by the Growth Hormone Research Society, areas of uncertainty include the safety of growth hormone in children with cancer-predisposing conditions, its use in growth hormone–deficient children who are receiving maintenance therapy, and the overall benefit-risk ratio in adult survivors.[80]
Disorders of LH and FSH
- Pubertal development can be adversely affected by cranial radiation therapy.
- Doses higher than 18 Gy can result in central precocious puberty, while doses higher than 30 Gy to 40 Gy may result in LH/FSH deficiency.[49]
Central precocious puberty
Prevalence and risk factors
- Central precocious puberty is among the most common hypothalamic-pituitary dysfunctions. The prevalence varies across studies because of study cohort composition and method of ascertainment.[3,81,82]
- In the SJMB03 study, 156 children and adolescents with medulloblastoma were treated with surgery, risk-adapted photon craniospinal irradiation, and dose-intensive chemotherapy.[54]
- The 5-year cumulative incidence of precocious puberty was 2.0%.
- Children with CNS tumors within or near the hypothalamic-pituitary axis (including those with neurofibromatosis type 1) or those treated with cranial radiation are most vulnerable.[3]
- In a study of 178 childhood brain tumor survivors (median follow-up, 6.6 years), precocious puberty developed in 12.2% of survivors, with a 5-year cumulative incidence of 4.0%.[3]
- Central precocious puberty has been reported in children receiving cranial irradiation in doses of 18 Gy or higher.[81,83,84]
- Hydrocephalus also increases the risk of central precocious puberty.[85]
Diagnosis
- Central precocious puberty is defined by the onset of pubertal development before age 8 years in girls and 9 years in boys as a result of the premature activation of the hypothalamic-pituitary-gonadal axis.
- Assessment of puberty cannot be performed using testicular volume measurements in boys exposed to chemotherapy or direct radiation to the testes, given the toxic effect of these treatments on germ cells and repercussions on gonadal size.[86]
- The staging of puberty in males within this population relies on the presence of other signs of virilization, such as the presence of pubic hair and the measurement of morning plasma testosterone and LH levels.[86]
Treatment and outcomes associated with central precocious puberty
- Aside from the adjustment and psychosocial challenges associated with early pubertal development, precocious puberty can lead to the rapid closure of the skeletal growth plates and short stature. This deleterious effect can be further potentiated by growth hormone deficiency.[81,86]
- The increased growth velocity induced by pubertal development can mask concurrent growth hormone deficiency with seemingly normal growth velocity; this occurrence may mislead care providers.[86]
- The impact of central precocious puberty on linear growth can be ascertained by assessing the degree of skeletal maturation (or bone age) using an x-ray of the left hand.[87]
- Delaying the progression of puberty relies on the use of various gonadotropin-releasing hormone agonist preparations. This approach has been shown to improve growth prospects, especially when other pituitary abnormalities, including growth hormone deficiency, are concurrently treated.[42,88]
LH/FSH deficiency
- LH/FSH deficiency (also referred to as hypogonadotropic hypogonadism) can manifest through pubertal delay (after age 13 years in girls and 14 years in boys), arrested puberty, or symptoms of decreased sex hormone production, depending on age and pubertal status at the time of diagnosis.
- The risk of LH/FSH deficiency is highest among patients treated with cranial radiation at doses greater than or equal to 30 Gy. LH/FSH deficiency following the exposure to lower doses can occur at delayed time points.[4]
- With higher doses of cranial radiation therapy (>35 Gy), deficiencies in LH/FSH can be seen, with a cumulative incidence of 10% to 20% at 5 to 10 years posttreatment.[4,85]
- The treatment of LH/FSH deficiency relies on sex-hormone replacement therapy adjusted to age and pubertal status.[42]
TSH deficiency
TSH deficiency (also referred to as central hypothyroidism) in survivors of childhood cancer can have profound clinical consequences and be underappreciated. Among survivors of head and neck tumors treated with radiation therapy potentially exposing the hypothalamic-pituitary region and the thyroid gland, hypothyroidism may result from TRH and/or TSH deficiency (central hypothyroidism), thyroid gland dysfunction (primary hypothyroidism), or a combination of central and primary causes.
Prevalence and risk factors
- The risk of TSH deficiency is highest among patients treated with cranial radiation at doses of 30 Gy or higher. TSH deficiency following the exposure to lower doses can occur at delayed time points.[4]
- The cumulative incidence of TSH deficiency was 23% (± 8%) among embryonal brain tumor survivors treated with risk-adapted CSI, conformal primary site irradiation, and high-dose chemotherapy. Radiation dose to the hypothalamus in excess of 42 Gy is associated with an increased risk of developing TSH deficiency (44% ± 19% for a dose of ≥42 Gy and 11% ± 8% for a dose of <42 Gy).[89]
- In a report of 156 children and adolescents with medulloblastoma who were treated with surgery, risk-adapted photon craniospinal irradiation, and dose-intensive chemotherapy between 2003 and 2013, the incidence of hypothyroidism was 48.4%.[54]
- In average-risk patients, hypothyroidism was associated with younger age (HR, 0.902; P = .0070), hypothalamus dose (HR, 1.039; P = .0273), and thyroid dose (HR, 1.070; P = .0263). The mean radiation therapy dose was 34.8 Gy (standard deviation [SD], 7.5) to the hypothalamus and 17.4 Gy (SD, 5.4) to the thyroid.
- In high-risk patients, hypothyroidism was associated with increased hypothalamus dose (HR, 1.068; P = .0671) and thyroid dose (HR, 1.050; P = .0515). The mean radiation therapy dose was 47.8 Gy (SD, 4.87) to the hypothalamus and 25.8 Gy (SD, 7.2) to the thyroid.
- Among medulloblastoma survivors treated with photon (median follow-up, 9.6 years) or proton (median follow-up, 3.8 years) radiation therapy, primary hypothyroidism developed in 12 of 54 patients (22%) after photon radiation therapy and 3 of 41 patients (7%) after proton radiation therapy (HR, 2.1; P = .27). Central hypothyroidism (TSH deficiency) developed in 13 of 54 patients (24%) after photon radiation therapy and 4 of 41 patients (10%) after proton radiation therapy (HR, 2.16, P = .18).[90]
- Among 118 medulloblastoma survivors (median follow-up, 5.6 years), the estimated cumulative incidence of hypothyroidism was 35.8% (95% CI, 26.7%–47.1%) by 5 years after radiation therapy.[18]
- The median time from the end of radiation therapy to the development of hypothyroidism was 2.1 years (range, 0.1–7.1 years) after proton radiation therapy and 2.9 years (range, 0.8–7.2 years) after photon radiation therapy.
- The rate of hypothyroidism (HR, 2.36; 95% CI, 1.11–5.02) was higher in patients treated with photon radiation therapy than in those treated with proton radiation therapy.
- Differences in hypothyroidism risk by radiation modality were largely attributed to higher rates of primary hypothyroidism after photon radiation therapy (HR, 4.61; 95% CI, 1.20–17.66).
- Patients with central hypothyroidism should undergo testing for other hypothalamic-pituitary deficiencies before thyroid replacement. Thyroid replacement can enhance the clearance of cortisol, which exacerbates any underlying adrenal insufficiency and contributes to the possibility of adrenal crisis.
- In a study of 189 children and young adults (aged <26 years) with brain tumors who were treated with proton radiation therapy, the 4-year actuarial rate of hypothyroidism was 20.1%. Ninety percent of the cases were related to central TSH deficiency.[17]
Clinical presentation and diagnosis
- Symptoms of central hypothyroidism (e.g., asthenia, edema, drowsiness, and skin dryness) may have a gradual onset and go unrecognized until thyroid replacement therapy is initiated.
- In addition to delayed puberty and slow growth, hypothyroidism may cause fatigue, dry skin, constipation, increased sleep requirement, and cold intolerance.
- Individuals with TSH deficiency usually have low plasma free T4 levels and either low or inappropriately normal TSH levels. Declining free T4 levels within the normal range may also be predictive of TSH deficiency. Clinicians should not hesitate to reassess survivors with this pattern of thyroid function, especially in the presence of symptoms of hypothyroidism.[91]
- Mixed primary and central hypothyroidism can also occur because of separate injuries to the thyroid gland and the hypothalamus (e.g., radiation injury to both structures), leading to mildly elevated TSH values despite the contribution of hypothalamic-pituitary deficits to hypothyroidism in affected individuals.
Management of TSH deficiency
- Thyroid hormone replacement therapy using levothyroxine represents the mainstay of treatment of TSH deficiency.
- The dose of levothyroxine needs to be adjusted solely using plasma free T4 levels. The levels of TSH are expected to remain low during therapy, given the central nature of this deficiency.
- Central hypothyroidism should not be treated before assessment of the functioning of other hypothalamic-pituitary deficiencies, because it could precipitate an adrenal crisis in patients with ACTH deficiency.
ACTH deficiency
Prevalence and risk factors
- ACTH deficiency is less common than other neuroendocrine deficits but should be suspected in patients who have a history of brain tumors (regardless of therapy modality), cranial radiation therapy, growth hormone deficiency, or central hypothyroidism.[42,89,92,93]
- ACTH deficiency develops almost exclusively in survivors previously exposed to hypothalamic-pituitary doses of 30 Gy or greater.
- The cumulative incidence of ACTH deficiency was 38% (± 6%) among embryonal brain tumor survivors treated with risk-adapted CSI, conformal primary site irradiation, and high-dose chemotherapy. ACTH status was not affected by the dose of radiation therapy administered to the hypothalamus (median, 42.2 Gy for no ACTH deficiency vs. 41.3 Gy for ACTH deficiency).[89]
- In a study of 189 children and young adults (aged <26 years) with brain tumors who were treated with proton radiation therapy, the 5-year actuarial rate of ACTH deficiency was 8%, occurring almost exclusively after ≥40 Gy to the hypothalamus and pituitary.[17]
- One report included 156 children and adolescents with medulloblastoma who were treated with surgery, risk-adapted photon craniospinal irradiation, and dose-intensive chemotherapy in the SJMB03 study. The 5-year cumulative incidence of adrenal insufficiency was 13.0%.[54] Adrenal insufficiency was associated with increased hypothalamus dose (HR, 1.112; P < .0001).
- Although uncommon, ACTH deficiency can occur in patients treated with intracranial radiation doses of less than 24 Gy and in patients with non-CNS tumors or hematological malignancies distant from the hypothalamic-pituitary region. The use of glucocorticoid therapy in treatment protocols may also be associated with steroid-induced adrenal insufficiency, which in some cases may be prolonged.[94,95,96]
- In a PENTEC report on ACTH deficiency (6 cohorts, 230 patients), the dose associated with a 50% risk was 61 Gy (95% CI, 44.7–119.4).[20] The risk of ACTH deficiency was 20% in children who received a mean dose of 34 Gy in 2-Gy fractions to the hypothalamus-pituitary axis.
Diagnosis and management
- The diagnosis of ACTH deficiency should be suspected when low plasma levels of morning cortisol are measured (a screening cortisol level collected at 8 AM that is 10 µg/dL or more is reassuring for ACTH sufficiency, whereas a value of 5 µg/dL or lower is suspicious for insufficiency).
- Confirmation is necessary using dynamic testing such as the low-dose ACTH stimulation test.[92]
- Because of the substantial risk of central adrenal insufficiency among survivors treated with cranial radiation doses exceeding 30 Gy to the hypothalamic-pituitary axis, endocrine monitoring with periodic dynamic testing as clinically indicated is recommended for this high-risk group.[42]
- Patients with partial ACTH deficiency may have only subtle symptoms unless they become ill.[42]
- Illness can disrupt these patients' usual homeostasis and cause a more severe, prolonged, or complicated course than expected. As in complete ACTH deficiency, incomplete or unrecognized ACTH deficiency can be life-threatening during concurrent illness.
- The treatment of ACTH deficiency relies on replacement with hydrocortisone, including stress dosing in situations of illness to adjust to the body's physiologically increased need for glucocorticoids.[42]
Hyperprolactinemia
- Hyperprolactinemia has been described in patients who received radiation therapy to the hypothalamus in doses higher than 50 Gy or who underwent surgery that disrupted the integrity of the pituitary stalk.[97]
- Primary hypothyroidism may lead to hyperprolactinemia as a result of hyperplasia of thyrotrophs and lactotrophs, presumably caused by TRH hypersecretion.
- The prolactin response to TRH is usually exaggerated in these patients.[50,98]
- Hyperprolactinemia may result in delayed puberty, galactorrhea, menstrual irregularities, loss of libido, hot flashes, infertility, and osteopenia. Hyperprolactinemia resulting from cranial radiation therapy is rarely symptomatic and is frequently associated with hypogonadism (both central and primary).
- Hyperprolactinemia rarely requires treatment.[99]
Table 11 summarizes pituitary gland late effects and the related health screenings.
Table 11. Pituitary Gland Late Effectsa
Predisposing Therapy |
Endocrine/Metabolic Effects |
Health Screening |
BMI = body mass index; FSH = follicle-stimulating hormone; LH = luteinizing hormone; T4 = thyroxine; TSH = thyroid-stimulating hormone. |
a Adapted from theChildren's Oncology Group Long-Term Follow-Up Guidelines for Survivors of Childhood, Adolescent, and Young Adult Cancers. |
b Testicular volume measurements are not reliable in the assessment of pubertal development in boys exposed to chemotherapy or direct radiation to the testes. |
c Appropriate only at diagnosis. TSH levels are not useful for follow-up during replacement therapy. |
Tumor or surgery affecting hypothalamus/pituitary. Radiation exposing hypothalamic-pituitary axis. |
Growth hormone deficiency |
Assessment of nutritional status |
Height, weight, BMI, Tanner stageb |
Tumor or surgery affecting hypothalamus/pituitary or optic pathways; hydrocephalus. Radiation exposing hypothalamic-pituitary axis. |
Precocious puberty |
Height, weight, BMI, Tanner stageb |
FSH, LH, estradiol, or testosterone levels |
Tumor or surgery affecting hypothalamus/pituitary. Radiation exposing hypothalamic-pituitary axis. |
Gonadotropin deficiency |
History: puberty, sexual function |
Examination: Tanner stageb |
FSH, LH, estradiol or testosterone levels |
Tumor or surgery affecting hypothalamus/pituitary. Radiation exposing hypothalamic-pituitary axis. |
Central adrenal insufficiency |
History: failure to thrive, anorexia, episodic dehydration, hypoglycemia, lethargy, unexplained hypotension |
Endocrine consultation for those with radiation dose ≥30 Gy |
Radiation exposing hypothalamic-pituitary axis. |
Hyperprolactinemia |
History/examination: galactorrhea |
Prolactin level |
Radiation exposing hypothalamic-pituitary axis. |
Overweight/obesity |
Height, weight, BMI |
Blood pressure test |
Components of metabolic syndrome (abdominal obesity, hypertension, dyslipidemia, impaired glucose metabolism) |
Fasting blood glucose level and lipid profile |
Tumor or surgery affecting hypothalamus/pituitary. Radiation exposing hypothalamic-pituitary axis. |
Central hypothyroidism |
TSHc free thyroxine (free T4) level |
Testis and Ovary
Testicular and ovarian hormonal functions are discussed in the Late Effects of the Reproductive System section of this summary.
Metabolic Syndrome
An increased risk of metabolic syndrome or its components has been observed among survivors of childhood cancer. The evidence for this outcome ranges from clinically manifested conditions that are self-reported by survivors to retrospectively assessed data in medical records and hospital registries to systematic clinical evaluations of clinically well-characterized cohorts. Studies have been limited by cohort selection and participation bias, heterogeneity in treatment approach, time since treatment, and method of ascertainment. Despite these limitations, compelling evidence indicates that metabolic syndrome is highly associated with cardiovascular events and mortality.
Definitions of metabolic syndrome are evolving but generally include a combination of central (abdominal) obesity with at least two of the following features:[100]
- Hypertension.
- Atherogenic dyslipidemia (elevated triglycerides, reduced high-density lipoprotein [HDL] cholesterol).
- Abnormal glucose metabolism (fasting hyperglycemia, hyperinsulinism, insulin resistance, diabetes mellitus type 2).
Evidence (prevalence of and risk factors for metabolic syndrome in childhood cancer survivors):
- French investigators evaluated the overall and age-specific prevalence of and risk factors for metabolic syndrome and its components among 650 adult survivors of childhood leukemia treated without HSCT.[101]
- The overall prevalence of the condition was 6.9%, with the following age-specific cumulative prevalence:
- 20 years—1.3%.
- 25 years—6.1%.
- 30 years—10.8%.
- 35 years—22.4%.
- The prevalence of individual components of the metabolic syndrome was as follows:
- Increased fasting glucose—5.8%.
- Increased triglycerides—11.7%.
- Increased abdominal circumference—16.7%.
- Decreased HDL cholesterol—26.8%.
- Increased blood pressure—36.7%.
- Clinical factors significantly predicting the risk of metabolic syndrome included male sex (OR, 2.64; 95% CI, 1.32–5.29), age at last evaluation (OR, 1.10; 95% CI, 1.04–1.17) and body mass index (BMI) at diagnosis (OR, 1.15; 95% CI, 1.01–1.32), but not cumulative steroid dose. Irradiated and nonirradiated patients exhibited different patterns of metabolic abnormalities, with more frequent abdominal obesity in irradiated patients and more frequent hypertension in nonirradiated patients.
- A study monitored 784 long-term childhood ALL survivors (median age, 31.7 years) for a median follow-up of 26.1 years.[102]
- The prevalence of metabolic syndrome was 33.6%, which was significantly higher than that in a cohort of age-, sex-, and race-matched controls (n = 777) from the National Health and Nutrition Examination Survey (RR, 1.43; 95% CI, 1.22–1.69).
- Risk factors associated with metabolic syndrome in this study included older age and past exposures to cranial radiation therapy.
- Components of the metabolic syndrome that were significantly more prevalent in ALL survivors than in controls included obesity, insulin resistance, hypertension, and decreased HDL levels.
- The French childhood leukemia survivors' cohort showed that the prevalence of metabolic syndrome was increased among childhood leukemia survivors (n = 1,025), relative to controls (n = 3,202).[103]
- Metabolic syndrome was found in 10.3% of patients (mean follow-up, 16.3 years) and 4.5% of controls (OR, 2.49; P < .001). Transplant patients treated with TBI had the highest risk (OR, 6.26; P < .001). Other treatment groups that also showed a higher risk than controls included patients treated with chemotherapy only. ORs were 1.68 (P = .005) after chemotherapy only, 2.32 (P = .002) after chemotherapy and cranial irradiation, and 2.18 (P = .057) after transplant without irradiation.
- Compared with controls, unique profiles were seen in survivors according to treatment. Patients who received TBI and developed metabolic syndrome had a smaller waist circumference than controls. In contrast, patients who were treated with cranial irradiation and developed metabolic syndrome had a larger waist circumference than controls. Additionally, TBI recipients had increased triglyceride levels, fasting glucose levels, and systolic blood pressure.
- In a prospective study of 164 long-term survivors of embryonal tumors treated with abdominal radiation therapy (median follow-up, 26 years), nephroblastoma (OR, 5.2) and neuroblastoma (OR, 6.5) survivors had more components of metabolic syndrome than did controls.[104]
- Compared with survivors who did not receive radiation therapy, survivors treated with abdominal irradiation had higher blood pressure, triglycerides, low-density lipoprotein cholesterol, and total fat percentage, which were assessed by dual-energy x-ray absorptiometry.
Lifestyle impact on modifiable risk factors
- Modifiable risk factors (such as diet, physical activity, and smoking habits) may impact the risk of developing metabolic syndrome in childhood cancer survivors.
- Several studies have provided support for the potential benefits of lifestyle modifications in reducing cardiovascular disease risk.[105,106,107]
Evidence (lifestyle modifications to reduce cardiovascular risk in childhood cancer survivors):
- Survivors participating in the SJLIFE study who were adherent to a heart-healthy lifestyle had a lower risk of metabolic syndrome.[105]
- Females (RR, 2.4; 95% CI, 1.7–3.3) and males (RR, 2.2; 95% CI, 1.6–3.0) in the cohort who did not follow recommended dietary and physical activity guidelines had a more than twofold excess risk of having clinical features of the metabolic syndrome.
- A CCSS investigation evaluated the impact of exercise on cardiovascular disease risk among survivors of Hodgkin lymphoma.[106]
- Vigorous exercise was associated with a lower risk of cardiovascular events in a dose-dependent manner, independent of cardiovascular risk profile and treatment.
- Survivors who were adherent to national vigorous-intensity exercise guidelines had a 51% reduction in the risk of any cardiovascular event compared with those not meeting the guidelines.
- Another CCSS investigation evaluated the association of exercise with mortality in adult survivors of childhood cancer.[107]
- After adjusting for chronic health conditions and treatment exposures, all-cause mortality was inversely associated with survivor-reported exercise quartiles (0, 3–6, 9–12, and 15–21 metabolic equivalent task [MET] hours/week).
- Survivors who endorsed recommended levels of vigorous exercise (≥9 MET hours/week) in early adulthood and those who increased exercise over 8 years had a lower risk of mortality.
Abnormal glucose metabolism
For individuals treated at a young age (age <11 years), abdominal radiation therapy and TBI are increasingly recognized as independent risk factors for diabetes mellitus in childhood cancer survivors.[2,108,109,110,111,112]
Evidence (risk factors for diabetes mellitus in childhood cancer survivors):
- A single-center cohort study of 532 long-term adult survivors (median follow-up, 17.9 years; median age, 25.6 years) observed the following:[109]
- Treatment, but not genetic variation, was strongly associated with the occurrence of the components of metabolic syndrome.
- Metabolic syndrome was more frequent in survivors who received cranial (23.3%, P = .002) or abdominal (23.4%, P = .009) radiation therapy than in survivors who did not receive radiation therapy (10.0%).
- A cross-sectional study evaluated cardiovascular risk factors and insulin resistance in a clinically heterogeneous cohort of 319 childhood cancer survivors 5 or more years since diagnosis and 208 sibling controls.[113]
- Insulin resistance was significantly higher in survivors treated with cisplatin plus cranial irradiation (92% brain tumors) and in those who received steroids but no cisplatin (most leukemia survivors), compared with siblings.
- Insulin resistance did not differ between survivors treated with surgery alone and siblings.
- Among survivors, analysis of individual chemotherapy agents failed to find associations with cardiovascular risk factors or insulin resistance.
- Compared with siblings, nearly all chemotherapeutic agents, when examined individually, seemed to be associated with a high cardiovascular risk profile, characterized by lower total lean body mass, higher percentage fat mass, and insulin resistance.
- In a European multicenter cohort of 2,520 childhood cancer survivors (median follow-up, 28 years), significant associations were found between diabetes mellitus and increasing doses of radiation therapy to the tail of the pancreas. This finding supports the contribution of radiation-induced islet cell injury to impairments of glucose homeostasis in this population.[110]
- A report from the CCSS compared 8,599 childhood cancer survivors with 2,936 randomly selected sibling controls, and adjusted for age, BMI, and several demographic factors.[114]
- The risk of diabetes mellitus was 1.8 times higher in survivors (95% CI, 1.3–2.5; P < .001).
- Significant associations were found between diabetes mellitus and young age at diagnosis (0–4 years), the use of alkylating agents, and abdominal radiation therapy or TBI.
- Survivors exposed to abdominal radiation had a 3.4-fold higher increased risk of diabetes (95% CI, 2.3–5.0; P < .001) when compared with siblings and after adjusting for BMI. Compared with siblings, survivors of neuroblastoma and Wilms tumor who were treated with abdominal radiation therapy had a 6.9-fold and 2.1-fold increased risk of diabetes, respectively. Individuals in these groups who had not been exposed to abdominal radiation therapy were not at increased risk.
- Survivors were significantly more likely to be receiving medication for hypertension, dyslipidemia, and/or diabetes mellitus than were sibling controls.
Table 12 summarizes metabolic syndrome late effects and the related health screenings.
Body Composition: Underweight, Overweight, and Obesity
- Childhood cancer survivors are at risk of experiencing abnormal body composition, which includes becoming underweight (BMI, <18.5), becoming overweight (BMI, >25.0 to BMI, <30.0), or being affected by obesity (BMI, ≥30.0).
- BMI at diagnosis has been identified as a significant predictor of being underweight or overweight at follow-up. This finding suggests that genetic or environmental factors contribute to the development or persistence of abnormal body composition.[115,116]
Underweight
- CCSS investigators identified treatment-related risk factors for being underweight, including TBI (females) or abdominal irradiation (males), use of alkylating agents, and use of anthracyclines.[116]
- Among a cohort of 893 Dutch childhood cancer survivors monitored for a median of almost 15 years, being underweight was linked to a high prevalence of moderate to extreme adverse health statuses and reports of a major medical condition.[115]
Overweight/obesity
- To date, patients with cancer who have an increased incidence of being affected by overweight and obesity are primarily ALL [115,117,118,119,120,121] and CNS tumor [40,97] survivors who were treated with cranial radiation therapy.[116,122]
- The development of overweight/obesity after cranial radiation therapy is multifactorial and includes the following:[119,123,124]
- Growth hormone deficiency.
- Leptin sensitivity.
- Reduced levels of physical activity and energy expenditure.
Evidence (risk factors for overweight/obesity):
- In the nationwide Dutch Childhood Cancer Survivor Study (DCCSS-LATER) cohort of adult survivors of childhood cancer (n = 2,338), investigators found that having excess body weight (BMI >2.5 kg/m2) occurred in almost one-half of all long-term survivors. This incidence was not increased compared with the general population.[125]
- Overweight incidence was higher in women aged 50 years and older, and morbid obesity incidence was higher in men aged 50 years and older.
- Factors such as having excess body weight at cancer diagnosis, receiving cranial irradiation, and having growth hormone deficiency were all associated with long-term overweight status. Corticosteroids were not associated with overweight status.
- Dual-energy x-ray absorptiometry–based classification identified overweight in an additional 30% of survivors, particularly those treated with abdominal irradiation, TBI, anthracyclines, and platinum chemotherapy.
- CCSS investigators reported the following independent risk factors for obesity in childhood cancer survivors including treatment, lifestyle, and medication use:[126]
- Cancer diagnosed at ages 5 to 9 years (RR, 1.12; 95% CI, 1.01–1.24).
- Abnormal physical functioning (RR, 1.19; 95% CI, 1.06–1.33).
- Hypothalamic-pituitary radiation dose of 20 Gy to 30 Gy (RR, 1.17; 95% CI, 1.05–1.3; P = .01).
- Specific antidepressant use of paroxetine (RR, 1.29; 95% CI, 1.08–1.54).
- Survivors who adhered to the U.S. Centers for Disease Control and Prevention guidelines for vigorous physical activity (RR, 0.90; 95% CI, 0.82–0.97; P = .01) and who had a medium amount of anxiety (RR, 0.86; 95% CI, 0.75–0.99; P = .04) had a lower risk of obesity.[126]
Body composition alterations after childhood ALL
Evidence (risk factors for body composition alterations):
- Moderate-dose cranial radiation therapy (18–24 Gy) among ALL survivors is associated with obesity, particularly in females treated at a young age.[117,119,127]
- Female adult survivors of childhood ALL who were treated with cranial radiation therapy of 24 Gy before age 5 years are four times more likely to be affected by obesity than are women who have not been treated for a cancer.[117]
- Women treated with 18 Gy to 24 Gy cranial radiation therapy before age 10 years have a substantially greater rate of increase in their BMI through their young adult years than do women who were treated for ALL with only chemotherapy or women in the general population.[119]
- These women also have a significantly increased visceral adiposity and associated insulin resistance.[128,129]
- Body composition alterations appear to be attenuated in males.
- A study of long-term male survivors of ALL (mean age, 29 years) observed significantly higher body adiposity than in age-matched controls, despite normal weight and BMI.[130]
- Potential indicators of increased adiposity included high leptin and low sex hormone–binding globulin levels.[130]
- Serum testicular endocrine markers (testosterone, FSH, or inhibin B) did not correlate with body adiposity.[130]
- ALL therapy regimens are associated with increases in BMI shortly after completion of therapy, and possibly with a higher risk of obesity in the long term.[120,121,131,132,133]
- Several studies have reported that survivors of childhood ALL treated with chemotherapy alone also exhibit long-term changes in body composition, with relative increases in body fat [129,134,135,136] and visceral adiposity in comparison to lean mass.[128]
- These changes cannot be detected if BMI alone is used in the assessment of metabolic risk in this population.
- A genetic predisposition to excess adiposity may be enhanced when a child with ALL is treated with cranial irradiation. Genetic determinants of BMI identified in the general population were investigated in two large cohorts of childhood cancer survivors (n = 1,458) (CCSS and SJLIFE study).[137]
- Among ALL survivors who were treated with cranial radiation therapy in childhood, investigators identified statistical interactions of cranial radiation therapy treatment in survivors with germline genetic variants known to affect the baseline genetic risk of adiposity. This suggests that a genetic predisposition to excess adiposity may be enhanced when an individual is treated with cranial radiation therapy for childhood ALL.
Evidence (body composition changes in adult survivors of childhood ALL):
- A cohort study of 365 adult survivors of ALL (149 treated with cranial radiation therapy and 216 treated without cranial radiation therapy) compared body composition, energy balance, and fitness with age-, sex-, and race-matched peers.[138]
- Female survivors who were not exposed to cranial irradiation had comparable body composition values to that of peers.
- Waist circumference, waist-to-height ratio, total fat mass, and percent fat mass were higher among male survivors and cranial radiation–exposed female survivors than among comparison group members.
- Survivors of both sexes exposed to cranial radiation therapy had higher BMI and percent body fat than did survivors not exposed to cranial radiation therapy.
- Although survivors who did not receive cranial radiation therapy had energy balances similar to the matched peer group, they had significantly higher measures of impaired fitness (impaired flexibility, peripheral sensorimotor deficits, proximal muscle weakness, and poor exercise tolerance).
- In a CCSS study that was based on self-reported height and weight measurements, the following was observed:
- Adult survivors of childhood ALL treated with chemotherapy alone did not have significantly higher rates of obesity than sibling controls.[117]
- There were also no differences in BMI changes between these groups after a subsequent period of follow-up that averaged 7.8 years.[119]
- Swiss investigators evaluated self-reported weight in 1,936 adult survivors of childhood ALL, non-Hodgkin lymphoma, and Hodgkin lymphoma (median age, 24 years; median time from diagnosis, 17 years) and compared them with siblings and the general population.[139]
- The proportion of survivors who reported being overweight (26%) was comparable to that of siblings (24%) and the general Swiss population (25%).
- There was no evidence of a relationship between cumulative glucocorticoid dose and being overweight.
- There was also no evidence that the use of cranial radiation therapy modified the effect of the cumulative glucocorticoid dose on being overweight.
Variable outcomes across studies likely relate to the use of BMI as the metric for abnormal body composition, which does not adequately assess visceral adiposity that can contribute to metabolic risk in this population.[140]
Body composition alterations after treatment for CNS tumors
Among brain tumor survivors treated with higher doses of cranial radiation therapy, the highest risk of developing obesity has been observed in females treated at a younger age.[141]
Survivors of craniopharyngioma have a substantially increased risk of developing extreme obesity because of the tumor location and the hypothalamic damage resulting from surgical resection.[142,143,144,145]
A cohort of 661 childhood survivors of brain tumors (mean follow-up time, 7.3 years) was evaluated for weight gain associated with hypothalamic-pituitary dysfunction. This cohort excluded survivors who had craniopharyngiomas or pituitary tumors.[146]
- Among survivors between the ages of 4 and 20 years at follow-up, 20.3% were classified as overweight and 8.5% had obesity, compared with 10.5% and 2.7%, respectively, in the general Dutch population of comparable age.
- Hypothalamic-pituitary dysfunction was more prevalent in survivors who are overweight or have obesity than in survivors of normal weight.
- BMI SDS at diagnosis, diagnosis of low-grade glioma, and history of diabetes insipidus and central precocious puberty were associated with weight gain, being overweight, or having obesity.
Body composition alterations after HSCT
- Survivors of childhood cancer treated with TBI in preparation for an allogeneic HSCT have increased measures of body fatness (percent fat) while often having a normal BMI.[111,147,148,149]
- Longitudinal decline in BMI related to substantial decrease in lean mass has been observed among survivors of hematological malignancies treated with allogeneic HSCT.[150]
- This finding was largely attributable to TBI conditioning and severity of chronic GVHD.[150]
Body composition alterations after solid malignancies
- Survivors of abdominal and pelvic solid tumors treated with radiation therapy are at risk of alterations in body composition and metabolic health that can adversely affect functional performance.
- Among 431 survivors of childhood abdominal or pelvic tumors (median attained age, 29.9 years), higher cumulative doses of abdominal and pelvic radiation therapy were associated with lower lean muscle mass in both males and females.[151]
- Compared with survivors who have normal (high) lean mass and normal (low) fat mass, survivors with normal lean mass and high fat mass had a significantly higher risk of insulin resistance, low HDL cholesterol, reduced quadriceps strength, and reduced distance covered in a 6-minute walk.[151]
Body composition and frailty
Young adult survivors of childhood cancer have a higher-than-expected prevalence of frailty, a phenotype characterized by low muscle mass, self-reported exhaustion, low energy expenditure, slow walking speed, and weakness.[152]
- Individuals are considered prefrail if they have two of these five characteristics and frail if they have three or more of these characteristics.[152]
- The frailty phenotype increases in prevalence with aging, and has been associated with excess risk of mortality and onset of chronic conditions.[152]
- In CCSS participants, the overall prevalence of frailty among survivors (6.4%) was three times higher than that of sibling controls (2.2%).[153]
- The highest prevalence of frailty was reported in survivors of CNS tumors (9.5%) and bone tumors (8.1%).
- Cranial radiation, pelvic radiation (≥34 Gy), and lung surgery were independent risk factors for frailty in the CCSS cohort.
- In SJLIFE study participants (median age at study entry, 30 years), frailty prevalence increased from 6.2% to 13.6% during the 5 years of follow-up.[154]
- Risk factors for frailty at follow-up included treatment with chest radiation therapy of 20 Gy or higher (OR, 1.98), cardiac (OR, 1.58) and neurological (OR, 2.58) conditions, lack of strength training (OR, 1.74), sedentary lifestyle (OR, 1.75), and frailty at study entry (OR, 11.12).
- Prior history of frailty was the strongest risk factor for death during follow-up (OR, 3.52).
- In an SJLIFE study (follow-up interval, 5 years), young-adult survivors of childhood cancer who are considered prefrail and frail (n = 205) experienced significant declines in neurocognitive domains commonly associated with dementia and Alzheimer disease.[155]
- Survivors considered frail declined more on tests of short-term verbal recall and executive function than did survivors not considered frail.
- Survivors considered frail and prefrail experienced greater declines in focused attention than did survivors not considered frail.
- The prevalence of and risk factors for prefrailty, frailty, and sarcopenia were evaluated in a DCCSS-LATER cross-sectional study of adult survivors (mean age, 33.1 years at participation) of childhood cancer who were treated between 1963 and 2001.[156]
- The prefrailty rate was 20.3%, the frailty rate was 7.4%, and the sarcopenia rate was 4.4%.
- Significant risk factors for prefrailty included the following:
- Being underweight (OR, 3.38; 95% CI, 1.92–5.95).
- Having obesity (OR, 1.67; 95% CI, 1.14–2.43).
- Exposure to cranial irradiation (OR, 2.07; 95% CI, 1.47–2.93).
- Exposure to TBI (OR, 3.17; 95% CI, 1.77–5.70).
- Exposure to cisplatin dose of at least 600 mg/m2 (OR, 3.75; 95% CI, 1.82–7.74).
- Having a growth hormone deficiency (OR, 2.25; 95% CI, 1.23–4.09).
- Having hyperthyroidism (OR, 3.72; 95% CI, 1.63–8.47).
- Low bone mineral density (OR for Z score ≤−1 and >-2, 1.80; 95% CI, 1.31–2.47 and OR for Z score ≤-2, 3.37; 95% CI, 2.20–5.15).
- Having a folic acid deficiency (OR, 1.87; 95% CI, 1.31–2.68).
- Significant risk factors for sarcopenia included the following:
- Male sex (OR, 4.56; 95% CI, 2.26–9.17).
- Having a lower BMI (continuous, OR, 0.52; 95% CI, 0.45–0.60).
- Exposure to cranial irradiation (OR, 3.87; 95% CI, 1.80–8.31).
- Exposure to TBI (OR, 4.52; 95% CI, 1.67–12.20).
- Having hypogonadism (OR, 3.96; 95% CI, 1.40–11.18).
- Having a growth hormone deficiency (OR, 4.66; 95% CI, 1.44–15.15).
- Having a vitamin B12 deficiency (OR, 6.26).
- Ongoing research aims to elucidate the pathophysiology of frailty and develop/test interventions to prevent or reverse this condition.
Table 13 summarizes body composition late effects and the related health screenings.
For endocrine and metabolic syndrome late effects information, including risk factors, evaluation, and health counseling, see the Children's Oncology Group Long-Term Follow-Up Guidelines for Survivors of Childhood, Adolescent, and Young Adult Cancers.
References:
-
Brignardello E, Felicetti F, Castiglione A, et al.: Endocrine health conditions in adult survivors of childhood cancer: the need for specialized adult-focused follow-up clinics. Eur J Endocrinol 168 (3): 465-72, 2013.
-
Mostoufi-Moab S, Seidel K, Leisenring WM, et al.: Endocrine Abnormalities in Aging Survivors of Childhood Cancer: A Report From the Childhood Cancer Survivor Study. J Clin Oncol 34 (27): 3240-7, 2016.
-
Clement SC, Schouten-van Meeteren AY, Boot AM, et al.: Prevalence and Risk Factors of Early Endocrine Disorders in Childhood Brain Tumor Survivors: A Nationwide, Multicenter Study. J Clin Oncol 34 (36): 4362-4370, 2016.
-
Chemaitilly W, Li Z, Huang S, et al.: Anterior hypopituitarism in adult survivors of childhood cancers treated with cranial radiotherapy: a report from the St Jude Lifetime Cohort study. J Clin Oncol 33 (5): 492-500, 2015.
-
González Briceño LG, Kariyawasam D, Samara-Boustani D, et al.: High Prevalence of Early Endocrine Disorders After Childhood Brain Tumors in a Large Cohort. J Clin Endocrinol Metab 107 (5): e2156-e2166, 2022.
-
Clement SC, van Rijn RR, van Eck-Smit BL, et al.: Long-term efficacy of current thyroid prophylaxis and future perspectives on thyroid protection during 131I-metaiodobenzylguanidine treatment in children with neuroblastoma. Eur J Nucl Med Mol Imaging 42 (5): 706-15, 2015.
-
Clement SC, van Eck-Smit BL, van Trotsenburg AS, et al.: Long-term follow-up of the thyroid gland after treatment with 131I-Metaiodobenzylguanidine in children with neuroblastoma: importance of continuous surveillance. Pediatr Blood Cancer 60 (11): 1833-8, 2013.
-
Bombardieri E, Giammarile F, Aktolun C, et al.: 131I/123I-metaiodobenzylguanidine (mIBG) scintigraphy: procedure guidelines for tumour imaging. Eur J Nucl Med Mol Imaging 37 (12): 2436-46, 2010.
-
Sharp SE, Trout AT, Weiss BD, et al.: MIBG in Neuroblastoma Diagnostic Imaging and Therapy. Radiographics 36 (1): 258-78, 2016 Jan-Feb.
-
Clement SC, Tytgat GAM, van Trotsenburg ASP, et al.: Thyroid function after diagnostic 123I-metaiodobenzylguanidine in children with neuroblastic tumors. Ann Nucl Med 36 (6): 579-585, 2022.
-
Verloop H, Louwerens M, Schoones JW, et al.: Risk of hypothyroidism following hemithyroidectomy: systematic review and meta-analysis of prognostic studies. J Clin Endocrinol Metab 97 (7): 2243-55, 2012.
-
Zatelli MC, Lamartina L, Meringolo D, et al.: Thyroid nodule recurrence following lobo-isthmectomy: incidence, patient's characteristics, and risk factors. J Endocrinol Invest 41 (12): 1469-1475, 2018.
-
Chemaitilly W, Li Z, Brinkman TM, et al.: Primary hypothyroidism in childhood cancer survivors: Prevalence, risk factors, and long-term consequences. Cancer 128 (3): 606-614, 2022.
-
Bölling T, Geisenheiser A, Pape H, et al.: Hypothyroidism after head-and-neck radiotherapy in children and adolescents: preliminary results of the "Registry for the Evaluation of Side Effects After Radiotherapy in Childhood and Adolescence" (RiSK). Int J Radiat Oncol Biol Phys 81 (5): e787-91, 2011.
-
Inskip PD, Veiga LHS, Brenner AV, et al.: Hypothyroidism after Radiation Therapy for Childhood Cancer: A Report from the Childhood Cancer Survivor Study. Radiat Res 190 (2): 117-132, 2018.
-
Sklar C, Whitton J, Mertens A, et al.: Abnormalities of the thyroid in survivors of Hodgkin's disease: data from the Childhood Cancer Survivor Study. J Clin Endocrinol Metab 85 (9): 3227-32, 2000.
-
Vatner RE, Niemierko A, Misra M, et al.: Endocrine Deficiency As a Function of Radiation Dose to the Hypothalamus and Pituitary in Pediatric and Young Adult Patients With Brain Tumors. J Clin Oncol 36 (28): 2854-2862, 2018.
-
Aldrich KD, Horne VE, Bielamowicz K, et al.: Comparison of hypothyroidism, growth hormone deficiency, and adrenal insufficiency following proton and photon radiotherapy in children with medulloblastoma. J Neurooncol 155 (1): 93-100, 2021.
-
Milano MT, Vargo JA, Yorke ED, et al.: Primary Hypothyroidism in Childhood Cancer Survivors Treated With Radiation Therapy: A PENTEC Comprehensive Review. Int J Radiat Oncol Biol Phys 119 (2): 482-493, 2024.
-
Wheeler G, Grassberger C, Samers J, et al.: Central Endocrine Complications Among Childhood Cancer Survivors Treated With Radiation Therapy: A PENTEC Comprehensive Review. Int J Radiat Oncol Biol Phys 119 (2): 457-466, 2024.
-
Gleeson HK, Darzy K, Shalet SM: Late endocrine, metabolic and skeletal sequelae following treatment of childhood cancer. Best Pract Res Clin Endocrinol Metab 16 (2): 335-48, 2002.
-
Inskip PD, Veiga LHS, Brenner AV, et al.: Hyperthyroidism After Radiation Therapy for Childhood Cancer: A Report from the Childhood Cancer Survivor Study. Int J Radiat Oncol Biol Phys 104 (2): 415-424, 2019.
-
Clausen CT, Hasle H, Holmqvist AS, et al.: Hyperthyroidism as a late effect in childhood cancer survivors - an Adult Life after Childhood Cancer in Scandinavia (ALiCCS) study. Acta Oncol 58 (2): 227-231, 2019.
-
Bhatti P, Veiga LH, Ronckers CM, et al.: Risk of second primary thyroid cancer after radiotherapy for a childhood cancer in a large cohort study: an update from the childhood cancer survivor study. Radiat Res 174 (6): 741-52, 2010.
-
Haddy N, El-Fayech C, Guibout C, et al.: Thyroid adenomas after solid cancer in childhood. Int J Radiat Oncol Biol Phys 84 (2): e209-15, 2012.
-
Ronckers CM, Sigurdson AJ, Stovall M, et al.: Thyroid cancer in childhood cancer survivors: a detailed evaluation of radiation dose response and its modifiers. Radiat Res 166 (4): 618-28, 2006.
-
Sigurdson AJ, Ronckers CM, Mertens AC, et al.: Primary thyroid cancer after a first tumour in childhood (the Childhood Cancer Survivor Study): a nested case-control study. Lancet 365 (9476): 2014-23, 2005 Jun 11-17.
-
van Santen HM, Tytgat GA, van de Wetering MD, et al.: Differentiated thyroid carcinoma after 131I-MIBG treatment for neuroblastoma during childhood: description of the first two cases. Thyroid 22 (6): 643-6, 2012.
-
Veiga LH, Lubin JH, Anderson H, et al.: A pooled analysis of thyroid cancer incidence following radiotherapy for childhood cancer. Radiat Res 178 (4): 365-76, 2012.
-
Kovalchik SA, Ronckers CM, Veiga LH, et al.: Absolute risk prediction of second primary thyroid cancer among 5-year survivors of childhood cancer. J Clin Oncol 31 (1): 119-27, 2013.
-
Vivanco M, Dalle JH, Alberti C, et al.: Malignant and benign thyroid nodules after total body irradiation preceding hematopoietic cell transplantation during childhood. Eur J Endocrinol 167 (2): 225-33, 2012.
-
Li Z, Franklin J, Zelcer S, et al.: Ultrasound surveillance for thyroid malignancies in survivors of childhood cancer following radiotherapy: a single institutional experience. Thyroid 24 (12): 1796-805, 2014.
-
Brignardello E, Felicetti F, Castiglione A, et al.: Ultrasound surveillance for radiation-induced thyroid carcinoma in adult survivors of childhood cancer. Eur J Cancer 55: 74-80, 2016.
-
Clement SC, Kremer LCM, Verburg FA, et al.: Balancing the benefits and harms of thyroid cancer surveillance in survivors of Childhood, adolescent and young adult cancer: Recommendations from the international Late Effects of Childhood Cancer Guideline Harmonization Group in collaboration with the PanCareSurFup Consortium. Cancer Treat Rev 63: 28-39, 2018.
-
Metzger ML, Howard SC, Hudson MM, et al.: Natural history of thyroid nodules in survivors of pediatric Hodgkin lymphoma. Pediatr Blood Cancer 46 (3): 314-9, 2006.
-
Francis GL, Waguespack SG, Bauer AJ, et al.: Management Guidelines for Children with Thyroid Nodules and Differentiated Thyroid Cancer. Thyroid 25 (7): 716-59, 2015.
-
Sanders JE, Hoffmeister PA, Woolfrey AE, et al.: Thyroid function following hematopoietic cell transplantation in children: 30 years' experience. Blood 113 (2): 306-8, 2009.
-
Cattoni A, Molinari S, Gaiero A, et al.: Thyroid Disorders Following Hematopoietic Stem Cell Transplantation in Childhood: Impact of Conditioning Regimen on Thyroid Dysfunction, Volume Changes, and Occurrence of Nodules. Transplant Cell Ther 28 (8): 506.e1-506.e12, 2022.
-
van Iersel L, Mulder RL, Denzer C, et al.: Hypothalamic-Pituitary and Other Endocrine Surveillance Among Childhood Cancer Survivors. Endocr Rev 43 (5): 794-823, 2022.
-
Gurney JG, Kadan-Lottick NS, Packer RJ, et al.: Endocrine and cardiovascular late effects among adult survivors of childhood brain tumors: Childhood Cancer Survivor Study. Cancer 97 (3): 663-73, 2003.
-
Hudson MM, Ness KK, Gurney JG, et al.: Clinical ascertainment of health outcomes among adults treated for childhood cancer. JAMA 309 (22): 2371-81, 2013.
-
Sklar CA, Antal Z, Chemaitilly W, et al.: Hypothalamic-Pituitary and Growth Disorders in Survivors of Childhood Cancer: An Endocrine Society Clinical Practice Guideline. J Clin Endocrinol Metab 103 (8): 2761-2784, 2018.
-
Ramelli GP, von der Weid N, Stanga Z, et al.: Suprasellar germinomas in childhood and adolescence: diagnostic pitfalls. J Pediatr Endocrinol Metab 11 (6): 693-7, 1998 Nov-Dec.
-
Vinchon M, Baroncini M, Leblond P, et al.: Morbidity and tumor-related mortality among adult survivors of pediatric brain tumors: a review. Childs Nerv Syst 27 (5): 697-704, 2011.
-
Fahrner B, Prosch H, Minkov M, et al.: Long-term outcome of hypothalamic pituitary tumors in Langerhans cell histiocytosis. Pediatr Blood Cancer 58 (4): 606-10, 2012.
-
Lawson SA, Horne VE, Golekoh MC, et al.: Hypothalamic-pituitary function following childhood brain tumors: Analysis of prospective annual endocrine screening. Pediatr Blood Cancer 66 (5): e27631, 2019.
-
Lebbink CA, Ringers TP, Schouten-van Meeteren AYN, et al.: Prevalence and risk factors of hypothalamic-pituitary dysfunction in infant and toddler childhood brain tumor survivors. Eur J Endocrinol 185 (4): 597-606, 2021.
-
Fraser O, Crowne E, Tacey M, et al.: Correlating measured radiotherapy dose with patterns of endocrinopathy: The importance of minimizing pituitary dose. Pediatr Blood Cancer 69 (11): e29847, 2022.
-
van Iersel L, Li Z, Srivastava DK, et al.: Hypothalamic-Pituitary Disorders in Childhood Cancer Survivors: Prevalence, Risk Factors and Long-Term Health Outcomes. J Clin Endocrinol Metab 104 (12): 6101-6115, 2019.
-
Darzy KH, Shalet SM: Hypopituitarism following radiotherapy. Pituitary 12 (1): 40-50, 2009.
-
Viana MB, Vilela MI: Height deficit during and many years after treatment for acute lymphoblastic leukemia in children: a review. Pediatr Blood Cancer 50 (2 Suppl): 509-16; discussion 517, 2008.
-
Merchant TE, Goloubeva O, Pritchard DL, et al.: Radiation dose-volume effects on growth hormone secretion. Int J Radiat Oncol Biol Phys 52 (5): 1264-70, 2002.
-
Merchant TE, Rose SR, Bosley C, et al.: Growth hormone secretion after conformal radiation therapy in pediatric patients with localized brain tumors. J Clin Oncol 29 (36): 4776-80, 2011.
-
Merchant TE, Wu S, Onar-Thomas A, et al.: Endocrinopathy After Treatment for Medulloblastoma: Results From the SJMB03 Trial of Risk-Adapted Radiation Therapy. Int J Radiat Oncol Biol Phys 116 (3): 560-568, 2023.
-
van Iersel L, van Santen HM, Potter B, et al.: Clinical impact of hypothalamic-pituitary disorders after conformal radiation therapy for pediatric low-grade glioma or ependymoma. Pediatr Blood Cancer 67 (12): e28723, 2020.
-
Sklar C, Mertens A, Walter A, et al.: Final height after treatment for childhood acute lymphoblastic leukemia: comparison of no cranial irradiation with 1800 and 2400 centigrays of cranial irradiation. J Pediatr 123 (1): 59-64, 1993.
-
Chow EJ, Friedman DL, Yasui Y, et al.: Decreased adult height in survivors of childhood acute lymphoblastic leukemia: a report from the Childhood Cancer Survivor Study. J Pediatr 150 (4): 370-5, 375.e1, 2007.
-
Vandecruys E, Dhooge C, Craen M, et al.: Longitudinal linear growth and final height is impaired in childhood acute lymphoblastic leukemia survivors after treatment without cranial irradiation. J Pediatr 163 (1): 268-73, 2013.
-
Browne EK, Zhou Y, Chemaitilly W, et al.: Changes in body mass index, height, and weight in children during and after therapy for acute lymphoblastic leukemia. Cancer 124 (21): 4248-4259, 2018.
-
Demoor-Goldschmidt C, Allodji RS, Journy N, et al.: Risk Factors for Small Adult Height in Childhood Cancer Survivors. J Clin Oncol 38 (16): 1785-1796, 2020.
-
Mizumoto M, Oshiro Y, Pan H, et al.: Height after photon craniospinal irradiation in pediatric patients treated for central nervous system embryonal tumors. Pediatr Blood Cancer 67 (10): e28617, 2020.
-
Ogilvy-Stuart AL, Clark DJ, Wallace WH, et al.: Endocrine deficit after fractionated total body irradiation. Arch Dis Child 67 (9): 1107-10, 1992.
-
Willi SM, Cooke K, Goldwein J, et al.: Growth in children after bone marrow transplantation for advanced neuroblastoma compared with growth after transplantation for leukemia or aplastic anemia. J Pediatr 120 (5): 726-32, 1992.
-
Wingard JR, Plotnick LP, Freemer CS, et al.: Growth in children after bone marrow transplantation: busulfan plus cyclophosphamide versus cyclophosphamide plus total body irradiation. Blood 79 (4): 1068-73, 1992.
-
Huma Z, Boulad F, Black P, et al.: Growth in children after bone marrow transplantation for acute leukemia. Blood 86 (2): 819-24, 1995.
-
Bernard F, Bordigoni P, Simeoni MC, et al.: Height growth during adolescence and final height after haematopoietic SCT for childhood acute leukaemia: the impact of a conditioning regimen with BU or TBI. Bone Marrow Transplant 43 (8): 637-42, 2009.
-
Chemaitilly W, Sklar CA: Endocrine complications of hematopoietic stem cell transplantation. Endocrinol Metab Clin North Am 36 (4): 983-98; ix, 2007.
-
Socié G, Salooja N, Cohen A, et al.: Nonmalignant late effects after allogeneic stem cell transplantation. Blood 101 (9): 3373-85, 2003.
-
Cohen A, Rovelli A, Bakker B, et al.: Final height of patients who underwent bone marrow transplantation for hematological disorders during childhood: a study by the Working Party for Late Effects-EBMT. Blood 93 (12): 4109-15, 1999.
-
Brennan BM, Shalet SM: Endocrine late effects after bone marrow transplant. Br J Haematol 118 (1): 58-66, 2002.
-
Sfeir JG, Kittah NEN, Tamhane SU, et al.: Diagnosis of GH Deficiency as a Late Effect of Radiotherapy in Survivors of Childhood Cancers. J Clin Endocrinol Metab 103 (8): 2785-2793, 2018.
-
Raman S, Grimberg A, Waguespack SG, et al.: Risk of Neoplasia in Pediatric Patients Receiving Growth Hormone Therapy--A Report From the Pediatric Endocrine Society Drug and Therapeutics Committee. J Clin Endocrinol Metab 100 (6): 2192-203, 2015.
-
Chemaitilly W, Robison LL: Safety of growth hormone treatment in patients previously treated for cancer. Endocrinol Metab Clin North Am 41 (4): 785-92, 2012.
-
Sklar CA, Mertens AC, Mitby P, et al.: Risk of disease recurrence and second neoplasms in survivors of childhood cancer treated with growth hormone: a report from the Childhood Cancer Survivor Study. J Clin Endocrinol Metab 87 (7): 3136-41, 2002.
-
Ergun-Longmire B, Mertens AC, Mitby P, et al.: Growth hormone treatment and risk of second neoplasms in the childhood cancer survivor. J Clin Endocrinol Metab 91 (9): 3494-8, 2006.
-
Patterson BC, Chen Y, Sklar CA, et al.: Growth hormone exposure as a risk factor for the development of subsequent neoplasms of the central nervous system: a report from the childhood cancer survivor study. J Clin Endocrinol Metab 99 (6): 2030-7, 2014.
-
Bogarin R, Steinbok P: Growth hormone treatment and risk of recurrence or progression of brain tumors in children: a review. Childs Nerv Syst 25 (3): 273-9, 2009.
-
Tamhane S, Sfeir JG, Kittah NEN, et al.: GH Therapy in Childhood Cancer Survivors: A Systematic Review and Meta-Analysis. J Clin Endocrinol Metab 103 (8): 2794-2801, 2018.
-
Thomas-Teinturier C, Oliver-Petit I, Pacquement H, et al.: Influence of growth hormone therapy on the occurrence of a second neoplasm in survivors of childhood cancer. Eur J Endocrinol 183 (4): 471-480, 2020.
-
Boguszewski MCS, Boguszewski CL, Chemaitilly W, et al.: Safety of growth hormone replacement in survivors of cancer and intracranial and pituitary tumours: a consensus statement. Eur J Endocrinol 186 (6): P35-P52, 2022.
-
Chemaitilly W, Merchant TE, Li Z, et al.: Central precocious puberty following the diagnosis and treatment of paediatric cancer and central nervous system tumours: presentation and long-term outcomes. Clin Endocrinol (Oxf) 84 (3): 361-71, 2016.
-
Armstrong GT, Whitton JA, Gajjar A, et al.: Abnormal timing of menarche in survivors of central nervous system tumors: A report from the Childhood Cancer Survivor Study. Cancer 115 (11): 2562-70, 2009.
-
Didcock E, Davies HA, Didi M, et al.: Pubertal growth in young adult survivors of childhood leukemia. J Clin Oncol 13 (10): 2503-7, 1995.
-
Shalet SM, Crowne EC, Didi MA, et al.: Irradiation-induced growth failure. Baillieres Clin Endocrinol Metab 6 (3): 513-26, 1992.
-
Gan HW, Phipps K, Aquilina K, et al.: Neuroendocrine Morbidity After Pediatric Optic Gliomas: A Longitudinal Analysis of 166 Children Over 30 Years. J Clin Endocrinol Metab 100 (10): 3787-99, 2015.
-
Chemaitilly W, Sklar CA: Endocrine complications in long-term survivors of childhood cancers. Endocr Relat Cancer 17 (3): R141-59, 2010.
-
Greulich WW, Pyle SI: Radiographic Atlas of Skeletal Development of Hand and Wrist. 2nd ed. Stanford University Press, 1959.
-
Gleeson HK, Stoeter R, Ogilvy-Stuart AL, et al.: Improvements in final height over 25 years in growth hormone (GH)-deficient childhood survivors of brain tumors receiving GH replacement. J Clin Endocrinol Metab 88 (8): 3682-9, 2003.
-
Laughton SJ, Merchant TE, Sklar CA, et al.: Endocrine outcomes for children with embryonal brain tumors after risk-adapted craniospinal and conformal primary-site irradiation and high-dose chemotherapy with stem-cell rescue on the SJMB-96 trial. J Clin Oncol 26 (7): 1112-8, 2008.
-
Bielamowicz K, Okcu MF, Sonabend R, et al.: Hypothyroidism after craniospinal irradiation with proton or photon therapy in patients with medulloblastoma. Pediatr Hematol Oncol 35 (4): 257-267, 2018.
-
van Iersel L, Xu J, Potter BS, et al.: Clinical Importance of Free Thyroxine Concentration Decline After Radiotherapy for Pediatric and Adolescent Brain Tumors. J Clin Endocrinol Metab 104 (11): 4998-5007, 2019.
-
Kazlauskaite R, Evans AT, Villabona CV, et al.: Corticotropin tests for hypothalamic-pituitary- adrenal insufficiency: a metaanalysis. J Clin Endocrinol Metab 93 (11): 4245-53, 2008.
-
Rose SR, Danish RK, Kearney NS, et al.: ACTH deficiency in childhood cancer survivors. Pediatr Blood Cancer 45 (6): 808-13, 2005.
-
Clement SC, Peeters RP, Ronckers CM, et al.: Intermediate and long-term adverse effects of radioiodine therapy for differentiated thyroid carcinoma--a systematic review. Cancer Treat Rev 41 (10): 925-34, 2015.
-
Follin C, Erfurth EM: Long-Term Effect of Cranial Radiotherapy on Pituitary-Hypothalamus Area in Childhood Acute Lymphoblastic Leukemia Survivors. Curr Treat Options Oncol 17 (9): 50, 2016.
-
Rensen N, Gemke RJ, van Dalen EC, et al.: Hypothalamic-pituitary-adrenal (HPA) axis suppression after treatment with glucocorticoid therapy for childhood acute lymphoblastic leukaemia. Cochrane Database Syst Rev 11 (11): CD008727, 2017.
-
Constine LS, Woolf PD, Cann D, et al.: Hypothalamic-pituitary dysfunction after radiation for brain tumors. N Engl J Med 328 (2): 87-94, 1993.
-
Constine LS, Rubin P, Woolf PD, et al.: Hyperprolactinemia and hypothyroidism following cytotoxic therapy for central nervous system malignancies. J Clin Oncol 5 (11): 1841-51, 1987.
-
Melmed S, Casanueva FF, Hoffman AR, et al.: Diagnosis and treatment of hyperprolactinemia: an Endocrine Society clinical practice guideline. J Clin Endocrinol Metab 96 (2): 273-88, 2011.
-
National Cholesterol Education Program (NCEP) Expert Panel on Detection, Evaluation, and Treatment of High Blood Cholesterol in Adults (Adult Treatment Panel III): Third Report of the National Cholesterol Education Program (NCEP) Expert Panel on Detection, Evaluation, and Treatment of High Blood Cholesterol in Adults (Adult Treatment Panel III) final report. Circulation 106 (25): 3143-421, 2002.
-
Saultier P, Auquier P, Bertrand Y, et al.: Metabolic syndrome in long-term survivors of childhood acute leukemia treated without hematopoietic stem cell transplantation: an L.E.A. study. Haematologica 101 (12): 1603-1610, 2016.
-
Nottage KA, Ness KK, Li C, et al.: Metabolic syndrome and cardiovascular risk among long-term survivors of acute lymphoblastic leukaemia - From the St. Jude Lifetime Cohort. Br J Haematol 165 (3): 364-74, 2014.
-
Oudin C, Berbis J, Bertrand Y, et al.: Prevalence and characteristics of metabolic syndrome in adults from the French childhood leukemia survivors' cohort: a comparison with controls from the French population. Haematologica 103 (4): 645-654, 2018.
-
van Waas M, Neggers SJ, Raat H, et al.: Abdominal radiotherapy: a major determinant of metabolic syndrome in nephroblastoma and neuroblastoma survivors. PLoS One 7 (12): e52237, 2012.
-
Smith WA, Li C, Nottage KA, et al.: Lifestyle and metabolic syndrome in adult survivors of childhood cancer: a report from the St. Jude Lifetime Cohort Study. Cancer 120 (17): 2742-50, 2014.
-
Jones LW, Liu Q, Armstrong GT, et al.: Exercise and risk of major cardiovascular events in adult survivors of childhood hodgkin lymphoma: a report from the childhood cancer survivor study. J Clin Oncol 32 (32): 3643-50, 2014.
-
Scott JM, Li N, Liu Q, et al.: Association of Exercise With Mortality in Adult Survivors of Childhood Cancer. JAMA Oncol 4 (10): 1352-1358, 2018.
-
Baker KS, Ness KK, Steinberger J, et al.: Diabetes, hypertension, and cardiovascular events in survivors of hematopoietic cell transplantation: a report from the bone marrow transplantation survivor study. Blood 109 (4): 1765-72, 2007.
-
van Waas M, Neggers SJ, Uitterlinden AG, et al.: Treatment factors rather than genetic variation determine metabolic syndrome in childhood cancer survivors. Eur J Cancer 49 (3): 668-75, 2013.
-
de Vathaire F, El-Fayech C, Ben Ayed FF, et al.: Radiation dose to the pancreas and risk of diabetes mellitus in childhood cancer survivors: a retrospective cohort study. Lancet Oncol 13 (10): 1002-10, 2012.
-
Armenian SH, Chemaitilly W, Chen M, et al.: National Institutes of Health Hematopoietic Cell Transplantation Late Effects Initiative: The Cardiovascular Disease and Associated Risk Factors Working Group Report. Biol Blood Marrow Transplant 23 (2): 201-210, 2017.
-
Lega IC, Pole JD, Austin PC, et al.: Diabetes Risk in Childhood Cancer Survivors: A Population-Based Study. Can J Diabetes 42 (5): 533-539, 2018.
-
Baker KS, Chow EJ, Goodman PJ, et al.: Impact of treatment exposures on cardiovascular risk and insulin resistance in childhood cancer survivors. Cancer Epidemiol Biomarkers Prev 22 (11): 1954-63, 2013.
-
Meacham LR, Chow EJ, Ness KK, et al.: Cardiovascular risk factors in adult survivors of pediatric cancer--a report from the childhood cancer survivor study. Cancer Epidemiol Biomarkers Prev 19 (1): 170-81, 2010.
-
van Santen HM, Geskus RB, Raemaekers S, et al.: Changes in body mass index in long-term childhood cancer survivors. Cancer 121 (23): 4197-204, 2015.
-
Meacham LR, Gurney JG, Mertens AC, et al.: Body mass index in long-term adult survivors of childhood cancer: a report of the Childhood Cancer Survivor Study. Cancer 103 (8): 1730-9, 2005.
-
Oeffinger KC, Mertens AC, Sklar CA, et al.: Obesity in adult survivors of childhood acute lymphoblastic leukemia: a report from the Childhood Cancer Survivor Study. J Clin Oncol 21 (7): 1359-65, 2003.
-
Sklar CA, Mertens AC, Walter A, et al.: Changes in body mass index and prevalence of overweight in survivors of childhood acute lymphoblastic leukemia: role of cranial irradiation. Med Pediatr Oncol 35 (2): 91-5, 2000.
-
Garmey EG, Liu Q, Sklar CA, et al.: Longitudinal changes in obesity and body mass index among adult survivors of childhood acute lymphoblastic leukemia: a report from the Childhood Cancer Survivor Study. J Clin Oncol 26 (28): 4639-45, 2008.
-
Chow EJ, Pihoker C, Hunt K, et al.: Obesity and hypertension among children after treatment for acute lymphoblastic leukemia. Cancer 110 (10): 2313-20, 2007.
-
Withycombe JS, Post-White JE, Meza JL, et al.: Weight patterns in children with higher risk ALL: A report from the Children's Oncology Group (COG) for CCG 1961. Pediatr Blood Cancer 53 (7): 1249-54, 2009.
-
Nathan PC, Jovcevska V, Ness KK, et al.: The prevalence of overweight and obesity in pediatric survivors of cancer. J Pediatr 149 (4): 518-25, 2006.
-
Oeffinger KC: Are survivors of acute lymphoblastic leukemia (ALL) at increased risk of cardiovascular disease? Pediatr Blood Cancer 50 (2 Suppl): 462-7; discussion 468, 2008.
-
Brennan BM, Rahim A, Blum WF, et al.: Hyperleptinaemia in young adults following cranial irradiation in childhood: growth hormone deficiency or leptin insensitivity? Clin Endocrinol (Oxf) 50 (2): 163-9, 1999.
-
Pluimakers VG, van Atteveld JE, de Winter DTC, et al.: Prevalence, risk factors, and optimal way to determine overweight, obesity, and morbid obesity in the first Dutch cohort of 2338 long-term survivors of childhood cancer: a DCCSS-LATER study. Eur J Endocrinol 189 (5): 495-507, 2023.
-
Green DM, Cox CL, Zhu L, et al.: Risk factors for obesity in adult survivors of childhood cancer: a report from the Childhood Cancer Survivor Study. J Clin Oncol 30 (3): 246-55, 2012.
-
Veringa SJ, van Dulmen-den Broeder E, Kaspers GJ, et al.: Blood pressure and body composition in long-term survivors of childhood acute lymphoblastic leukemia. Pediatr Blood Cancer 58 (2): 278-82, 2012.
-
Janiszewski PM, Oeffinger KC, Church TS, et al.: Abdominal obesity, liver fat, and muscle composition in survivors of childhood acute lymphoblastic leukemia. J Clin Endocrinol Metab 92 (10): 3816-21, 2007.
-
Oeffinger KC, Adams-Huet B, Victor RG, et al.: Insulin resistance and risk factors for cardiovascular disease in young adult survivors of childhood acute lymphoblastic leukemia. J Clin Oncol 27 (22): 3698-704, 2009.
-
Jahnukainen K, Heikkinen R, Henriksson M, et al.: Increased Body Adiposity and Serum Leptin Concentrations in Very Long-Term Adult Male Survivors of Childhood Acute Lymphoblastic Leukemia. Horm Res Paediatr 84 (2): 108-15, 2015.
-
Dalton VK, Rue M, Silverman LB, et al.: Height and weight in children treated for acute lymphoblastic leukemia: relationship to CNS treatment. J Clin Oncol 21 (15): 2953-60, 2003.
-
Kohler JA, Moon RJ, Wright S, et al.: Increased adiposity and altered adipocyte function in female survivors of childhood acute lymphoblastic leukaemia treated without cranial radiation. Horm Res Paediatr 75 (6): 433-40, 2011.
-
Zhang FF, Rodday AM, Kelly MJ, et al.: Predictors of being overweight or obese in survivors of pediatric acute lymphoblastic leukemia (ALL). Pediatr Blood Cancer 61 (7): 1263-9, 2014.
-
Warner JT, Evans WD, Webb DK, et al.: Body composition of long-term survivors of acute lymphoblastic leukaemia. Med Pediatr Oncol 38 (3): 165-72, 2002.
-
Miller TL, Lipsitz SR, Lopez-Mitnik G, et al.: Characteristics and determinants of adiposity in pediatric cancer survivors. Cancer Epidemiol Biomarkers Prev 19 (8): 2013-22, 2010.
-
Jarfelt M, Lannering B, Bosaeus I, et al.: Body composition in young adult survivors of childhood acute lymphoblastic leukaemia. Eur J Endocrinol 153 (1): 81-9, 2005.
-
Richard MA, Brown AL, Belmont JW, et al.: Genetic variation in the body mass index of adult survivors of childhood acute lymphoblastic leukemia: A report from the Childhood Cancer Survivor Study and the St. Jude Lifetime Cohort. Cancer 127 (2): 310-318, 2021.
-
Ness KK, DeLany JP, Kaste SC, et al.: Energy balance and fitness in adult survivors of childhood acute lymphoblastic leukemia. Blood 125 (22): 3411-9, 2015.
-
Belle FN, Kasteler R, Schindera C, et al.: No evidence of overweight in long-term survivors of childhood cancer after glucocorticoid treatment. Cancer 124 (17): 3576-3585, 2018.
-
Lindemulder SJ, Stork LC, Bostrom B, et al.: Survivors of standard risk acute lymphoblastic leukemia do not have increased risk for overweight and obesity compared to non-cancer peers: a report from the Children's Oncology Group. Pediatr Blood Cancer 62 (6): 1035-41, 2015.
-
Gurney JG, Ness KK, Stovall M, et al.: Final height and body mass index among adult survivors of childhood brain cancer: childhood cancer survivor study. J Clin Endocrinol Metab 88 (10): 4731-9, 2003.
-
Sahakitrungruang T, Klomchan T, Supornsilchai V, et al.: Obesity, metabolic syndrome, and insulin dynamics in children after craniopharyngioma surgery. Eur J Pediatr 170 (6): 763-9, 2011.
-
Müller HL: Childhood craniopharyngioma: current controversies on management in diagnostics, treatment and follow-up. Expert Rev Neurother 10 (4): 515-24, 2010.
-
Simoneau-Roy J, O'Gorman C, Pencharz P, et al.: Insulin sensitivity and secretion in children and adolescents with hypothalamic obesity following treatment for craniopharyngioma. Clin Endocrinol (Oxf) 72 (3): 364-70, 2010.
-
Tosta-Hernandez PDC, Siviero-Miachon AA, da Silva NS, et al.: Childhood Craniopharyngioma: A 22-Year Challenging Follow-Up in a Single Center. Horm Metab Res 50 (9): 675-682, 2018.
-
van Schaik J, van Roessel IMAA, Schouten-van Meeteren NAYN, et al.: High Prevalence of Weight Gain in Childhood Brain Tumor Survivors and Its Association With Hypothalamic-Pituitary Dysfunction. J Clin Oncol 39 (11): 1264-1273, 2021.
-
Neville KA, Cohn RJ, Steinbeck KS, et al.: Hyperinsulinemia, impaired glucose tolerance, and diabetes mellitus in survivors of childhood cancer: prevalence and risk factors. J Clin Endocrinol Metab 91 (11): 4401-7, 2006.
-
Nysom K, Holm K, Michaelsen KF, et al.: Degree of fatness after allogeneic BMT for childhood leukaemia or lymphoma. Bone Marrow Transplant 27 (8): 817-20, 2001.
-
Ketterl TG, Chow EJ, Leisenring WM, et al.: Adipokines, Inflammation, and Adiposity in Hematopoietic Cell Transplantation Survivors. Biol Blood Marrow Transplant 24 (3): 622-626, 2018.
-
Inaba H, Yang J, Kaste SC, et al.: Longitudinal changes in body mass and composition in survivors of childhood hematologic malignancies after allogeneic hematopoietic stem-cell transplantation. J Clin Oncol 30 (32): 3991-7, 2012.
-
Wilson CL, Liu W, Chemaitilly W, et al.: Body Composition, Metabolic Health, and Functional Impairment among Adults Treated for Abdominal and Pelvic Tumors during Childhood. Cancer Epidemiol Biomarkers Prev 29 (9): 1750-1758, 2020.
-
Ness KK, Krull KR, Jones KE, et al.: Physiologic frailty as a sign of accelerated aging among adult survivors of childhood cancer: a report from the St Jude Lifetime cohort study. J Clin Oncol 31 (36): 4496-503, 2013.
-
Hayek S, Gibson TM, Leisenring WM, et al.: Prevalence and Predictors of Frailty in Childhood Cancer Survivors and Siblings: A Report From the Childhood Cancer Survivor Study. J Clin Oncol 38 (3): 232-247, 2020.
-
Delaney A, Howell CR, Krull KR, et al.: Progression of Frailty in Survivors of Childhood Cancer: A St. Jude Lifetime Cohort Report. J Natl Cancer Inst 113 (10): 1415-1421, 2021.
-
Williams AM, Krull KR, Howell CR, et al.: Physiologic Frailty and Neurocognitive Decline Among Young-Adult Childhood Cancer Survivors: A Prospective Study From the St Jude Lifetime Cohort. J Clin Oncol 39 (31): 3485-3495, 2021.
-
van Atteveld JE, de Winter DTC, Pluimakers VG, et al.: Frailty and sarcopenia within the earliest national Dutch childhood cancer survivor cohort (DCCSS-LATER): a cross-sectional study. Lancet Healthy Longev 4 (4): e155-e165, 2023.
Late Effects of the Immune System
Late effects of the immune system have not been well studied, especially in survivors treated with contemporary therapies. Reports published about long-term immune system outcomes are limited by retrospective data collection, small sample size, cohort selection and participation bias, heterogeneity in treatment approach, time since treatment, and method of ascertainment.
Asplenia
Surgical or functional splenectomy increases the risk of life-threatening invasive bacterial infection:[1]
- Although staging laparotomy is no longer standard practice for pediatric Hodgkin lymphoma, patients from earlier treatment periods have ongoing risks.[2]
- Children may be rendered asplenic by radiation therapy to the spleen in doses greater than 30 Gy.[3,4] Low-dose involved-field radiation therapy (21 Gy) combined with multiagent chemotherapy did not appear to adversely affect splenic function, as measured by pitted red blood cell assays.[4] No other studies of immune status after radiation therapy are available.
- Functional asplenia (with Howell-Jolly bodies, reduced splenic size and blood flow) after hematopoietic stem cell transplant (HSCT) has been attributed to graft-versus-host disease (GVHD).[5]
- Childhood Cancer Survivor Study investigators observed a significantly increased risk of late infection-related mortality among survivors who were treated with splenectomy (relative risk [RR], 7.7; 95% confidence interval [CI], 3.1–19.1).[6]
- Splenic radiation was also associated with a dose-related risk of late infection-related mortality (0.1–9.9 Gy: RR, 2.0; 95% CI, 0.9–4.5; 10.0–19.9 Gy: RR, 5.5; 95% CI, 1.9–15.4; >20.0 Gy: RR, 6.0; 95% CI, 1.8–20.2).
- The low cumulative incidence of infection-related late mortality of 1.5% at 35 years after splenectomy and 0.6% after splenic radiation indicates that these are rare events.
- A Dutch multicenter cohort study included survivors of Hodgkin lymphoma (n = 4,919; age, <51 years at treatment; median follow-up, 20.2 years) who were treated between 1965 and 2000.[7]
- The study found that infectious-disease mortality was more than twofold increased, not only among patients who underwent splenectomy, but also in patients who received radiation therapy to the spleen.
- Infection-related mortality was slightly more increased after spleen irradiation than after splenectomy. This observation was thought to be related to the lower vaccination rate among patients who received spleen irradiation compared with patients who had a splenectomy.
Individuals with asplenia, regardless of the reason for the asplenic state, have an increased risk of fulminant bacteremia, especially associated with encapsulated bacteria, which is associated with a high mortality rate.[8]
The risk of bacteremia is higher in younger children than in older children, and this risk may be higher during the years immediately after splenectomy. Fulminant septicemia, however, has been reported in adults up to 25 years after splenectomy.
Bacteremia may be caused by the following organisms in asplenic survivors:
- Streptococcus pneumoniae. The most common pathogen that causes bacteremia in children with asplenia.
- Other streptococci.
- Haemophilus influenzae type b (Hib).
- Neisseria meningitidis.
- Escherichia coli; Staphylococcus aureus.
- Gram-negative bacilli, such as the Salmonella species, the Klebsiella species, and Pseudomonas aeruginosa.
Individuals with functional or surgical asplenia are also at increased risk of fatal malaria and severe babesiosis.[9]
Posttherapy management
- Clinicians should consider and encourage the administration of inactivated vaccines (e.g., influenza) and vaccines made of purified antigens (e.g., pneumococcus), bacterial components (e.g., diphtheria-tetanus-pertussis), or genetically engineered recombinant antigens (e.g., hepatitis B) in all cancer and transplant survivors according to recommended doses and schedules.[10,11,12]
- Two primary doses of quadrivalent meningococcal conjugate vaccine should be administered 2 months apart to children with asplenia, from age 2 years through adolescence, and a booster dose should be administered every 5 years. For more information, see Meningococcal Vaccination: Recommendations of the Advisory Committee on Immunization Practices, United States, 2020.[13]
- The efficacy of meningococcal vaccines in children with asplenia has not been established.
- No known contraindication exists to giving these vaccines at the same time as other required vaccines, in separate syringes, and at different sites.
- Pneumococcal conjugate vaccine (PCV) and pneumococcal polysaccharide vaccine (PPSV) are indicated at the recommended age for all children with asplenia.[14,15]
- Following the administration of the appropriate number of doses of PCV13, PPSV23 should be administered starting at age 24 months.
- A second dose should be administered 5 years later.
- For children aged 2 to 5 years with a complete PCV7 series who have not received PCV13, a supplemental dose of PCV13 should be administered.
- For asplenic individuals aged 6 to 18 years who have not received a dose of PCV13, a supplemental dose of PCV13 should be considered.
- Hib immunization should be initiated at age 2 months, as recommended for otherwise healthy young children and for previously unimmunized children with asplenia.[15] For more information, see Immunization Schedules.[15]
Daily antimicrobial prophylaxis against pneumococcal infections is recommended for young children with asplenia, regardless of their immunization status.
- Although the efficacy of daily antimicrobial prophylaxis has been proven only in patients with sickle cell anemia, this experience has been extended to other high-risk children, including asplenic children with a history of malignant neoplasms or thalassemia.
- In general, antimicrobial prophylaxis (in addition to immunization) should be considered for all children with asplenia younger than 5 years and for at least 1 year after splenectomy.
- The age at which antimicrobial prophylaxis is discontinued is an empiric decision.
- On the basis of a multicenter study in sickle cell disease, prophylactic penicillin can be discontinued at age 5 years among those who are receiving regular medical attention and who have not had a severe pneumococcal infection or surgical splenectomy.
- The appropriate duration of prophylaxis is unknown for children with asplenia attributable to other causes.
- Some experts continue prophylaxis throughout childhood and into adulthood for particularly high-risk patients with asplenia.
Table 14 summarizes spleen late effects and the related health screenings.
Table 14. Spleen Late Effectsa
Predisposing Therapy |
Immunologic Effects |
Health Screening/Interventions |
GVHD = graft-versus-host disease; HSCT = hematopoietic stem cell transplant; IgA = immunoglobulin A; T = temperature. |
a Adapted from theChildren's Oncology Group Long-Term Follow-Up Guidelines for Survivors of Childhood, Adolescent, and Young Adult Cancers. |
Radiation exposing spleen; splenectomy; HSCT with currently active GVHD |
Asplenia/hyposplenia; overwhelming post-splenectomy sepsis |
Blood cultures during febrile episodes (T >38.5°C); empiric antibiotics |
Immunization for encapsulated organisms (pneumococcal,Haemophilus influenzaetype b, and meningococcal vaccines) |
HSCT with any history of chronic GVHD |
Immunologic complications (secretory IgA deficiency, hypogammaglobulinemia, decreased B cells, T cell dysfunction, chronic infections [e.g., conjunctivitis, sinusitis, and bronchitis associated with chronic GVHD]) |
History: chronic conjunctivitis, chronic sinusitis, chronic bronchitis, recurrent or unusual infections, sepsis |
Examination: attention to eyes, nose/sinuses, and lungs |
For more information about posttransplant immunization, see the Centers for Disease Control and Prevention (CDC) Guidelines for Preventing Opportunistic Infections Among Hematopoietic Stem Cell Transplant RecipientsGuidelines for Preventing Opportunistic Infections Among Hematopoietic Stem Cell Transplant Recipients.
Humoral Immunity
Although the immune system appears to recover from the effects of active chemotherapy and radiation therapy, its effect on immune function into survivorship has not been well studied. Most studies have evaluated children treated for acute lymphoblastic leukemia (ALL), which in the disease itself, involves impaired lymphocyte maturation of either T or B cells in addition to lymphotoxic therapy.[16] Small studies suggest that protective titers against vaccine-preventable illnesses can be affected for months to years after non-HSCT childhood cancer therapy. There are no universal standards for the timing of serological evaluations or whether boosters and revaccinations should be given to every survivor.[16]
- Defects in immune recovery characterized by B-cell depletion have been observed in 2-year survivors of standard-risk and intermediate-risk ALL.[17]
- In a retrospective study of 55 childhood cancer survivors who received standard chemotherapy, more than 75% of long-term survivors who received at least one measles, mumps, and rubella (MMR) vaccination before their cancer diagnosis lacked seroprotection to the measles and mumps viruses. This suggests that the interrupted schedule is not sufficient for long-term seroprotection. All childhood cancer survivors who received both doses of the MMR vaccine after completion of their cancer treatment had positive titers against the measles and mumps viruses.[18]
- Survivors of childhood cancer may remain susceptible to vaccine-preventable infections. Treatment intensity, age at diagnosis, and time from treatment are associated with the risk of losing preexisting immunity.[19,20]
While there is a paucity of data regarding the benefits of administering active immunizations in this population, reimmunization is necessary to provide protective antibodies. The recommended reimmunization schedule will depend on previously received vaccinations and on the intensity of therapy.[16]
- In some children who received intensive treatment, consideration may be given to evaluating the antibodies against common vaccination antigens to determine the need for revaccination. For more information, see Immunization Schedules.[15]
Immune status is also compromised after HSCT, particularly in association with GVHD.[21]
- In a prospective longitudinal study of 210 survivors treated with allogeneic HSCT, antibody responses lasting for more than 5 years after immunization were observed in most patients for tetanus (95.7%), rubella (92.3%), poliovirus (97.9%), and, in diphtheria-tetanus-acellular pertussis (DTaP) recipients, diphtheria (100%).[22]
- Responses to pertussis (25.0%), measles (66.7%), mumps (61.5%), hepatitis B (72.9%), and diphtheria in tetanus-diphtheria (Td) recipients (48.6%) were less favorable.
- Factors associated with vaccine failure include older age at immunization; lower CD3, CD4, or CD19 count; higher immunoglobulin (Ig) M concentration; positive recipient cytomegalovirus serology; negative titer before immunization; history of acute or chronic GVHD; and radiation conditioning.
The major North American and European transplant groups, the CDC, and the Infectious Diseases Society of America have published follow-up recommendations for transplant recipients.[23,24,25] The American Society for Transplantation and Cellular Therapy has provided recommendations for preventing measles in patients who received HSCT or chimeric antigen receptor (CAR) T-cell therapy.[26]
Late effects after CD19-targeted CAR T-cell therapy for patients with relapsed or refractory ALL are largely unknown. Many patients will undergo an HSCT after CAR-T cell therapy, which complicates assessment of late effects. The most frequent late effect (as defined as >90 days post CAR T-cell therapy) is hypogammaglobulinemia.
- In a series of 75 children who received CAR T-cell therapy, all patients who responded to treatment (n = 61) experienced B-cell aplasia. There was an 83% probability of ongoing B-cell aplasia at 6 months after treatment with tisagenlecleucel. Ninety percent of responders to CAR T-cell therapy required intravenous (IV) IgG.[27]
- One series included 86 adults (young adults aged 22 years and older were included) who were treated with CAR T cells for CD19-positive refractory or relapsed conditions (ALL, chronic lymphocytic leukemia, and non-Hodgkin lymphoma) and alive for at least 1 year after treatment. Late effects were observed starting or persisting beyond 90 days after CAR T-cell therapy. Hypogammaglobulinemia (IgG <400mg/dL or IV gamma globulin replacement) was observed in 67% of patients with available data.[28]
Preexisting humoral immunity to vaccine-related antigens can persist in patients despite marked B-cell aplasia after CD19-targeted T-cell immunotherapy. Studies have shown that a population of plasma cells lacking CD19 expression survives long-term after CD19 CAR T-cell immunotherapy.[29]
Infection Risks
Infectious complications resulting in hospitalization occur in excess rates among long-term childhood cancer survivors.[30]
- In a retrospective, population-based, observational study, the incidence rate was 12.6 per 1,000 person-years for infection associated with hospitalization among 5-year childhood cancer survivors, compared with 2.4 for individuals without cancer who were matched for birth year and sex. This represented an incidence rate ratio of 5.1.
- Incident rates remained elevated more than twofold during the 5- to 10-year and longer than 10-year postdiagnosis periods.
- Survivors had a greater risk of infection (incident rate ratio, 13.1) relative to comparators when potentially vaccine-preventable infections were evaluated.
For immune system late effects information, including risk factors, evaluation, and health counseling, see the Children's Oncology Group Long-Term Follow-Up Guidelines for Survivors of Childhood, Adolescent, and Young Adult Cancers.
References:
-
Centers for Disease Control and Prevention: Altered immunocompetence. In: Kroger A, Bahta L, Long S, et al.: General Best Practice Guidelines for Immunization: Best Practices Guidance. Atlanta, GA: Advisory Committee on Immunization Practices (ACIP); Centers for Disease Control and Prevention, 2023, pp 123-48. Available online. Last accessed November 3, 2023.
-
Jockovich M, Mendenhall NP, Sombeck MD, et al.: Long-term complications of laparotomy in Hodgkin's disease. Ann Surg 219 (6): 615-21; discussion 621-4, 1994.
-
Coleman CN, McDougall IR, Dailey MO, et al.: Functional hyposplenia after splenic irradiation for Hodgkin's disease. Ann Intern Med 96 (1): 44-7, 1982.
-
Weiner MA, Landmann RG, DeParedes L, et al.: Vesiculated erythrocytes as a determination of splenic reticuloendothelial function in pediatric patients with Hodgkin's disease. J Pediatr Hematol Oncol 17 (4): 338-41, 1995.
-
Al-Eid MA, Tutschka PJ, Wagner HN, et al.: Functional asplenia in patients with chronic graft-versus-host disease: concise communication. J Nucl Med 24 (12): 1123-6, 1983.
-
Weil BR, Madenci AL, Liu Q, et al.: Late Infection-Related Mortality in Asplenic Survivors of Childhood Cancer: A Report From the Childhood Cancer Survivor Study. J Clin Oncol 36 (16): 1571-1578, 2018.
-
de Vries S, Schaapveld M, Janus CPM, et al.: Long-Term Cause-Specific Mortality in Hodgkin Lymphoma Patients. J Natl Cancer Inst 113 (6): 760-769, 2021.
-
Madenci AL, Armstrong LB, Kwon NK, et al.: Incidence and risk factors for sepsis after childhood splenectomy. J Pediatr Surg 54 (7): 1445-1448, 2019.
-
Ram S, Lewis LA, Rice PA: Infections of people with complement deficiencies and patients who have undergone splenectomy. Clin Microbiol Rev 23 (4): 740-80, 2010.
-
National Center for Immunization and Respiratory Diseases: General recommendations on immunization: recommendations of the Advisory Committee on Immunization Practices (ACIP). MMWR Morb Mortal Wkly Rep 60 (RR02): 1-60, 2011. Available online. Last accessed August 21, 2023.
-
Bridges CB, Coyne-Beasley T; Advisory Committee on Immunization Practices: Advisory committee on immunization practices recommended immunization schedule for adults aged 19 years or older: United States, 2014. Ann Intern Med 160 (3): 190, 2014.
-
Rubin LG, Levin MJ, Ljungman P, et al.: 2013 IDSA clinical practice guideline for vaccination of the immunocompromised host. Clin Infect Dis 58 (3): 309-18, 2014.
-
Mbaeyi SA, Bozio CH, Duffy J, et al.: Meningococcal Vaccination: Recommendations of the Advisory Committee on Immunization Practices, United States, 2020. MMWR Morb Mortal Wkly Rep 69 (No. RR-9): 1–41, 2020. Available online. Last accessed November 3, 2023.
-
Centers for Disease Control and Prevention (CDC): Use of 13-valent pneumococcal conjugate vaccine and 23-valent pneumococcal polysaccharide vaccine among children aged 6-18 years with immunocompromising conditions: recommendations of the Advisory Committee on Immunization Practices (ACIP). MMWR Morb Mortal Wkly Rep 62 (25): 521-4, 2013.
-
Centers for Disease Control and Prevention: Child and Adolescent Immunization Schedule by Medical Indication: Recommendations for Ages 18 Years or Younger, United States, 2023. Atlanta, GA: Centers for Disease Control and Prevention, 2023. Available online. Last accessed November 3, 2023.
-
Guilcher GMT, Rivard L, Huang JT, et al.: Immune function in childhood cancer survivors: a Children's Oncology Group review. Lancet Child Adolesc Health 5 (4): 284-294, 2021.
-
Koskenvuo M, Ekman I, Saha E, et al.: Immunological Reconstitution in Children After Completing Conventional Chemotherapy of Acute Lymphoblastic Leukemia is Marked by Impaired B-cell Compartment. Pediatr Blood Cancer 63 (9): 1653-6, 2016.
-
Speckhart SA: MMR vaccination timing and long-term immunity among childhood cancer survivors. Pediatr Blood Cancer 70 (4): e30133, 2023.
-
Fayea NY, Fouda AE, Kandil SM: Immunization status in childhood cancer survivors: A hidden risk which could be prevented. Pediatr Neonatol 58 (6): 541-545, 2017.
-
Bochennek K, Allwinn R, Langer R, et al.: Differential loss of humoral immunity against measles, mumps, rubella and varicella-zoster virus in children treated for cancer. Vaccine 32 (27): 3357-61, 2014.
-
Olkinuora HA, Taskinen MH, Saarinen-Pihkala UM, et al.: Multiple viral infections post-hematopoietic stem cell transplantation are linked to the appearance of chronic GVHD among pediatric recipients of allogeneic grafts. Pediatr Transplant 14 (2): 242-8, 2010.
-
Inaba H, Hartford CM, Pei D, et al.: Longitudinal analysis of antibody response to immunization in paediatric survivors after allogeneic haematopoietic stem cell transplantation. Br J Haematol 156 (1): 109-17, 2012.
-
Tomblyn M, Chiller T, Einsele H, et al.: Guidelines for preventing infectious complications among hematopoietic cell transplantation recipients: a global perspective. Biol Blood Marrow Transplant 15 (10): 1143-238, 2009.
-
Cordonnier C, Einarsdottir S, Cesaro S, et al.: Vaccination of haemopoietic stem cell transplant recipients: guidelines of the 2017 European Conference on Infections in Leukaemia (ECIL 7). Lancet Infect Dis 19 (6): e200-e212, 2019.
-
Majhail NS, Rizzo JD, Lee SJ, et al.: Recommended screening and preventive practices for long-term survivors after hematopoietic cell transplantation. Rev Bras Hematol Hemoter 34 (2): 109-33, 2012.
-
Pergam SA, Englund JA, Kamboj M, et al.: Preventing Measles in Immunosuppressed Cancer and Hematopoietic Cell Transplantation Patients: A Position Statement by the American Society for Transplantation and Cellular Therapy. Biol Blood Marrow Transplant 25 (11): e321-e330, 2019.
-
Maude SL, Laetsch TW, Buechner J, et al.: Tisagenlecleucel in Children and Young Adults with B-Cell Lymphoblastic Leukemia. N Engl J Med 378 (5): 439-448, 2018.
-
Cordeiro A, Bezerra ED, Hirayama AV, et al.: Late Events after Treatment with CD19-Targeted Chimeric Antigen Receptor Modified T Cells. Biol Blood Marrow Transplant 26 (1): 26-33, 2020.
-
Bhoj VG, Arhontoulis D, Wertheim G, et al.: Persistence of long-lived plasma cells and humoral immunity in individuals responding to CD19-directed CAR T-cell therapy. Blood 128 (3): 360-70, 2016.
-
Chehab L, Doody DR, Esbenshade AJ, et al.: A Population-Based Study of the Long-Term Risk of Infections Associated With Hospitalization in Childhood Cancer Survivors. J Clin Oncol 41 (2): 364-372, 2023.
Late Effects of the Musculoskeletal System
The musculoskeletal system of growing children and adolescents is vulnerable to the cytotoxic effects of cancer therapies, including surgery, chemotherapy, and radiation therapy. Documented late effects include the following:
- Bone and joint (abnormal bone and/or muscle growth) problems.
- Deformity and functional loss associated with amputation/limb-sparing surgery, joint contracture, osteoporosis/fractures, and osteonecrosis.
- Changes in body composition (obesity and loss of lean muscle mass).
While these late effects are discussed individually, it is important to remember that the components of the musculoskeletal system are interrelated. For example, hypoplasia of a muscle group can negatively affect the function of the long bones and the resultant dysfunction can subsequently lead to disuse and osteoporosis.
The major strength of the published literature documenting musculoskeletal late effects among children and adolescents treated for cancer is that most studies have clearly defined outcomes and exposures. However, many studies are observational and cross-sectional or retrospective in design. Single-institution studies are common, and for some outcomes, only small convenience cohorts have been described. Thus, it is possible that studies either excluded patients with the most severe musculoskeletal effects because of death or inability to participate in follow-up testing, or they oversampled those with the most severe musculoskeletal late effects because these patients were accessible as they returned for complication-related follow-up. Additionally, some of the results reported in adult survivors of childhood cancer may not be relevant to patients currently being treated because the anticancer treatment and delivery of anticancer modalities, particularly radiation therapy, have changed over the years in response to documented toxicities.
Abnormal Bone Growth
The effect of radiation on bone growth depends on the sites irradiated, as follows:
- Head and brain.
- Spine and long bones.
Radiation to the head and brain
In an age- and dose-dependent fashion, radiation can inhibit normal bone and muscle maturation and development.
- Radiation to the head (e.g., cranial, orbital, infratemporal, or nasopharyngeal radiation therapy) can cause craniofacial abnormalities, particularly in children treated before age 5 years who received radiation doses of 20 Gy or higher [1,2,3,4] or who were treated with concomitant chemotherapy.[5]
- Soft tissue sarcomas such as orbital rhabdomyosarcoma and retinoblastoma are two of the more common cancer types treated with these radiation fields. Often, in addition to the cosmetic impact of the craniofacial abnormalities, there can be related dental and sinus problems.
Cranial radiation therapy damages the hypothalamic-pituitary axis in an age- and dose-response fashion and can result in growth hormone deficiency.[6,7] If the growth hormone deficiency is not treated during the growing years and, sometimes, even with appropriate treatment, it leads to a substantially lower final height. Patients with a central nervous system (CNS) tumor [8,9] or acute lymphoblastic leukemia (ALL) [10,11] treated with 18 Gy or higher of cranial radiation therapy are at highest risk. Patients treated with single-fraction total-body irradiation (TBI),[12,13,14] and those treated with cranial radiation for non-CNS solid tumors [15] are also at risk of growth hormone deficiency. If the spine is also irradiated (e.g., craniospinal radiation therapy for medulloblastoma or early ALL therapies in the 1960s), growth can be affected by two separate mechanisms—growth hormone deficiency and direct damage to the spine.
Radiation to the spine and long bones
Radiation therapy can also directly affect the growth of the spine and long bones (and associated muscle groups) and can cause premature closure of the epiphyses, leading to the following:[16,17,18]
- Short stature.
- Asymmetric growth (scoliosis/kyphosis).
- Limb-length discrepancy.
Orthovoltage radiation therapy, commonly used before 1970, delivered high doses of radiation to bone and was commonly associated with subsequent abnormalities in bone growth.
However, even with contemporary radiation therapy, if a solid tumor is located near an epiphysis or the spine, alterations in normal bone development can be difficult to avoid.
The effects of radiation therapy administered to the spine on survivors of CNS tumors and Wilms tumor have been assessed.
Evidence (effect of radiation therapy on the spine and long bones):
- The effect of craniospinal irradiation (CSI) on bone growth was assessed in 220 pediatric patients with brain tumors who were treated with CSI.[19]
- Vertebral growth was significantly correlated with radiation dose (>39 Gy) and growth hormone deficiency.
- Eighty-three percent of patients had fatty marrow changes, 31% had disc degenerative changes (signal change, bulging, and height loss), 13% had degenerative changes of spinal bones, 17% had wedging or spinal height loss, and 27% had scoliosis.
- Even with symmetrical irradiation to the vertebral bodies, the risk of scoliosis was high after CSI.
- Age at irradiation was a significant risk factor for scoliosis (P = .0067), but there was no correlation with radiation dose.
- Adequate growth hormone replacement was important for bone growth.
- In the National Wilms Tumor Study (studies 1 through 4), stature loss in 2,778 children was evaluated.[20]
- For those younger than 12 months at diagnosis who received more than 10 Gy of radiation therapy, the estimated adult-height deficit was 7.7 cm when compared with the nonradiation therapy group.
- For those who received 10 Gy, the estimated trunk shortening was 2.8 cm or less.
- Among those whose height measurements in the teenage years were available, patients who received more than 15 Gy of radiation therapy were 4 to 7 cm shorter on average than their nonirradiated counterparts, with an evident dose-response relationship.
- Chemotherapy did not confer additional risk.
- The effect of radiation therapy on the development of scoliosis has also been re-evaluated. In a group of 42 children treated for Wilms tumor from 1968 to 1994, scoliosis was seen in 18 patients, with only one patient needing orthopedic intervention. The study also suggested that the incidence was lower in patients who received 10 to 12 Gy, which are the dosages currently used for Wilms tumor. However, the sample size was small.[21]
- In a cohort of 245 long-term survivors of ALL (median follow-up, 15.1 years), the prevalence of and risk factors for vertebral deformities were assessed by spine radiographs and dual-energy x-ray absorptiometry scans.[22]
- Vertebral deformities were prevalent in 23% of patients, with 88% of them categorized as Genant grade 1 (>20%–25% reduction in percentage of anterior, middle, or posterior vertebral height ratios).
- Independent predictors of prevalent vertebral deformity included male sex (relative risk [RR], 1.94; 95% CI, 1.16–3.24), higher cumulative glucocorticoid dose (RR, 1.05; 95% CI, 1.00–1.10), and back pain (RR, 2.44; 95% CI, 1.56–3.84).
- Lumbar spine bone mineral density Z-score and age at and time from diagnosis were not significant predictors of vertebral deformity. However, sex-stratified analyses indicated that lumbar spine Z-score was significantly predictive of vertebral deformity in males.
Osteoporosis and Fractures
Although increased rates of fracture are not reported among long-term survivors of childhood cancer,[23] maximal peak bone mass is an important factor influencing the risk of osteoporosis and fracture among older patients. Treatment-related factors that affect bone mineral loss include the following:
-
Chemotherapy. Methotrexate has a cytotoxic effect on osteoblasts, resulting in a reduction of bone volume and formation of new bone.[24] This effect may be exacerbated by the chronic use of corticosteroids, another class of agents routinely used in the treatment of hematological malignancies and in supportive care for a variety of pediatric cancers.
-
Radiation therapy. Radiation-related endocrinopathies, such as growth hormone deficiency or hypogonadism, may contribute to ongoing bone mineral loss.[25,26]
-
Nutrition and activity. Suboptimal nutrition and physical inactivity may further predispose to deficits in bone mineral accretion.
Most of our knowledge about cancer and treatment effects on bone mineralization has been derived from studies of children with ALL.
- In children with ALL, the leukemic process, and possibly vitamin D deficiency, may play a role in the alterations in bone metabolism and bone mass observed at diagnosis.[27]
- Antileukemic therapy causes additional bone mineral density loss,[28] which has been reported to normalize over time [29] or to persist for many years after completion of therapy.[30]
- Clinical factors predicting higher risk of low bone mineral density include treatment with the following:[31,32,33]
- High cumulative doses of methotrexate (>40 g/m2).
- High cumulative doses of corticosteroids (>9 g/m2).
- Cranial radiation therapy or craniospinal radiation therapy.
- More potent glucocorticoids such as dexamethasone.
- The development of osteonecrosis during treatment for ALL.[34]
Clinical assessment of bone mineral density in adults treated for childhood ALL indicates that most bone mineral deficits normalize over time after discontinuing osteotoxic therapy.[32]
Evidence (low bone mineral density):
- Dutch Childhood Oncology Group (DCOG)–LATER investigators evaluated the risk factors for low and very low bone mineral density, any fractures, and vertebral fractures with dual-energy x-ray absorptiometry in 3,996 5-year survivors of childhood cancer (mean age, 33 years at participation). Self-reported fractures were assessed among all participants, and vertebral fractures were assessed among a subset of participants.[26]
- The standardized incidence ratio of any first fracture was 3.53 (95% confidence interval [CI], 3.06–4.06) for male participants and 5.35 (4.46–6.52) for female participants. Thirty-three of 249 (13.3%) participants had vertebral fractures.
- Risk factors for low bone mineral density at any site included male sex, being underweight, exposure to high-dose carboplatin, exposure to any dose of cranial radiation therapy, hypogonadism, hyperthyroidism, low physical activity, and severe vitamin D deficiency.
- Risk factors for very low bone marrow density at any site included male sex, being underweight, exposure to cranial radiation therapy, growth hormone deficiency, and severe vitamin D deficiency.
- Risk factors for any fracture included male sex, former and current smoking status, and very low lumbar spine bone mineral density.
- Risk factors for vertebral fractures included older age at follow-up, previous treatment with platinum compounds, growth hormone deficiency, and low physical activity.
- Bone mineral density deficits have been reported in long-term survivors of childhood brain tumors.[35]
- Among 50 survivors of medulloblastoma or CNS supratentorial primitive neuroectodermal tumors (median follow-up, 19.5 years), decreased bone marrow density z-scores were detected in 48% of the lumbar spine and 34% of the total body measurements.
- Modifiable risk factors for bone marrow density deficits included low serum calcium (62%) and vitamin D (26%), low vitamin D intake (69%), and inactive lifestyle (62%).
- Male sex, older age at diagnosis, and lower lean body mass index (BMI) were associated with increased risk of decreased bone marrow density z-scores.
- Among 862 ALL survivors (median age, 31.3 years) evaluated by quantitative computed tomography of L1 through L2 vertebrae, the following was reported:[25]
- 30% of survivors had low bone mineral density (z-score below -1), and 18.6% of survivors met criteria for frailty or prefrailty.
- The prefrail phenotype is characterized by having two of five characteristics (low muscle mass, self-reported exhaustion, low energy expenditure, slow walking speed, and weakness), and the frail phenotype is characterized by having three or more of these characteristics.
- Modifiable factors such as growth hormone deficiency, smoking, and alcohol consumption were significant predictors for these outcomes, with varying impact on the basis of sex.
- These data underscore the importance of lifestyle counseling and screening for hormonal deficits during long-term survivors' follow-up evaluations.
- A cohort of 845 adult survivors of childhood ALL were evaluated at a median age of 31 years.[32]
- Very low bone mineral density was relatively uncommon, with only 5.7% of patients with bone mineral density z-scores consistent with osteoporosis and 23.8% of patients with bone mineral density z-scores consistent with osteopenia.
- Cranial radiation dose of 24 Gy or higher, but not cumulative methotrexate or prednisone equivalent doses, was associated with a twofold elevated risk of bone mineral density z-scores of -1 or lower.
- In a subset of 400 survivors with longitudinal bone mineral density evaluations, bone mineral density z-scores tended to improve from adolescence to young adulthood.
- Bone mineral density deficits that are likely multifactorial in etiology have been reported in allogeneic hematopoietic stem cell transplant (HSCT) recipients conditioned with TBI.
- French investigators observed a significant risk of lower femoral bone mineral density among adult survivors of childhood leukemia treated with HSCT who had gonadal deficiency.[36]
- Hormonal therapy has been shown to enhance the bone mineral density of adolescent girls diagnosed with hypogonadism after HSCT.[37]
- A cross-sectional study evaluated the prevalence of and risk factors for vitamin D deficiency (<20 ng/mL) and its association with bone mineral density in 446 long-term childhood cancer survivors (median age, 27.5 years; median time from completion of therapy, 14.2 years).[38]
- Vitamin D deficiency was present in 24% of survivors.
- Independent predictors of vitamin D deficiency included high BMI (odds ratio [OR], 1.78 for patients who are overweight; OR, 2.4 for patients with obesity) and Hispanic or Black race and ethnicity (OR, 2.4).
- Survivors with vitamin D deficiency had a more-than-threefold risk (OR, 3.58) of reduced bone mineral density.
Fracture risk in childhood cancer survivors
- Despite disease-related and treatment-related risks of bone mineral density deficits, the prevalence of self-reported fractures among Childhood Cancer Survivor Study (CCSS) participants was lower than that reported by sibling controls. Predictors of increased prevalence of fracture by multivariable analyses included the following:[23]
- Among female survivors, increasing age at follow-up, White race, methotrexate treatment, and balance difficulties.
- Among male survivors, smoking history and White race.
- Radiation-induced fractures can occur with doses of radiation of 50 Gy or higher, which are often used to treat Ewing sarcoma of the extremity.[39]
Risk prediction model for bone mineral density deficits
Data from the St. Jude Life (SJLIFE) cohort study (development) and Erasmus Medical Center (validation) in the Netherlands were used to develop and validate prediction models for low and very low bone mineral density on the basis of clinical and treatment characteristics that identify adult survivors of childhood cancer who require screening by dual-energy x-ray absorptiometry.[40]
- Low bone mineral density was defined as lumbar spine bone mineral density and/or total-body bone mineral density z-score of -1 or lower; very low bone mineral density was defined as a z-score of -2 or lower.
- Low bone mineral density was present in 51% of SJLIFE study participants and 45% of Dutch participants, represented by survivors of both hematologic and solid malignancies. Very low bone mineral density was present in 20% of SJLIFE study participants and 10% of Dutch participants.
- The model, which included male sex, height, weight, attained age, current smoking status, and cranial irradiation, showed good performance for predicting risk of low bone mineral density (areas under the curve of 0.72 in the SJLIFE cohort and 0.69 in the Dutch cohort).
- The model, which included male sex, height, weight, attained age, cranial irradiation, and abdominal irradiation, showed good performance for predicting risk of very low bone mineral density (areas under the curve of 0.76 in the SJLIFE cohort and 0.75 in the Dutch cohort).
- These models correctly identified bone mineral density status in most White adult survivors through age 40 years using easily measured patient and treatment characteristics.
- The calculator to determine the predicted probability of low and very low bone mineral density for an individual survivor is available online.
Osteonecrosis
Osteonecrosis (also known as aseptic or avascular necrosis) is a rare, but well-recognized skeletal complication observed predominantly in survivors of pediatric hematological malignancies treated with corticosteroids.[41] The condition is characterized by death of one or more segments of bone that most often affects weight-bearing joints, especially the hips and knees.
- The prevalence of osteonecrosis has varied from 1% to 22% based on the study population, treatment protocol, method of evaluation, and time from treatment.[41,42,43,44,45,46,47]
- Longitudinal cohort studies have identified a spectrum of clinical manifestations of osteonecrosis, ranging from asymptomatic, spontaneously resolving imaging changes to painful progressive articular collapse requiring joint replacement.[48]
- Symptomatic osteonecrosis characterized by pain, joint swelling, and reduced mobility typically presents during the first 2 years of therapy, particularly in patients with ALL.
- These symptoms may improve over time, persist, or progress in the years after completion of therapy.[49] In one series, 60% of patients continued to have symptoms at a median follow-up of 4.9 years after diagnosis of osteonecrosis.[50]
- Impaired physical performance and reduced quality of life have been observed in long-term survivors of childhood leukemia and lymphoma (mean age, 28 years) with osteonecrosis.[51]
- Surgical procedures, including core decompression, osteotomy, and joint replacements, are sometimes performed in patients with persistently severe symptoms.[50] Percutaneous autologous mesenchymal stem cells have also been used for the treatment of ankle osteonecrosis in childhood and adolescent leukemia survivors.[52]
Factors that increase the risk of osteonecrosis include the following:
Exposure to corticosteroids and, possibly, methotrexate and concurrent asparaginase
- The most important treatment factor associated with the development of osteonecrosis is prolonged exposure to corticosteroids, which is typical in treatment regimens used for ALL, non-Hodgkin lymphoma, and HSCT.[46,47,48,53,54]
- Osteonecrosis risk may be related to type of corticosteroid, with some studies in patients with ALL indicating increased risk with the use of dexamethasone compared with prednisone.[55,56]
- Corticosteroid dosing schedule also appears to impact the risk of developing osteonecrosis. In the Children's Oncology Group (COG)-1961 trial for newly diagnosed high-risk ALL, patients were randomly assigned to receive either continuous (daily) dexamethasone or an alternate-week schedule of dexamethasone during the delayed intensification phase. The alternate-week schedule was associated with a lower incidence of osteonecrosis.[41]
- In addition to corticosteroids, exposure to methotrexate and concurrent asparaginase may contribute to the development of osteonecrosis.
Development of thromboembolism during antileukemic therapy
- In a retrospective review of 208 children treated for ALL, investigators at McMaster University reported a 5.21-fold (95% CI, 1.82–14.91) increased odds of osteonecrosis among children who experienced thromboembolism during antileukemic therapy than among those who did not have a thromboembolism, even after accounting for age and asparaginase exposure.[57]
HSCT conditioning and course
- In a large case-control study that evaluated risk factors for osteonecrosis using data from the Center for International Blood and Marrow Transplant Research, lower risks of osteonecrosis were seen in patients with nonmalignant diseases and in those who had received reduced-intensity conditioning regimens for malignant diseases than were seen in patients receiving myeloablative regimens for malignant diseases.[58]
- Several studies have reported an increased risk of osteonecrosis in association with chronic graft-versus-host disease (GVHD).[44,53,58]
Age at time of diagnosis or transplant
- Several studies have demonstrated that age at diagnosis (or at time of transplant) is a significant independent predictor of osteonecrosis.[41,46,55]
- Osteonecrosis is significantly more common in older children and adolescents than in younger children. In the COG-1961 trial for high-risk ALL, the 5-year cumulative incidence of symptomatic osteonecrosis was 1.0% for patients aged 1 to 9 years, 9.9% for patients aged 10 to 15 years, and 20% for patients aged 16 to 21 years (P < .0001).[41]
Race
- Osteonecrosis occurs more frequently in White patients than in Black patients.[54,59]
Genetic factors
- Genetic factors influencing folate metabolism, glucocorticoid metabolism, and adipogenesis have been linked to excess risk of osteonecrosis among survivors.[54,60,61]
- Two candidate gene studies indicate that children homozygous for a 28–base pair repeat within the 5' untranslated region of the TYMS gene are at increased risk of osteonecrosis.[54,61] This gene is associated with folate production and replacement and is inhibited by methotrexate.
- St. Jude Children's Research Hospital investigators observed an almost sixfold (OR, 5.6; 95% CI, 2.7–11.3) risk of osteonecrosis among survivors with polymorphism of the ACP1 gene, which regulates lipid levels and osteoblast differentiation.[45]
- Genome-wide association studies have identified potential risk variants in the BMP7, PROX1::AS1 fusion, GRID2 (children younger than 10 years), and GRIN3A genes, which are all associated with glucocorticoid receptor activity.[60,62]
Sex
- Studies evaluating the influence of sex on the risk of osteonecrosis have yielded conflicting results, with some suggesting a higher incidence in females [50,59,63] that has not been confirmed by others.[64]
Adolescent and young adults (AYA)
Chronic comorbidities were assessed in a cohort of 6,778 2-year survivors of AYA cancer (defined as diagnosed with cancer between the ages of 15 and 39 years), with a median follow-up of 5.1 years after cancer diagnosis. The risk of developing chronic comorbidities was compared with matched individuals without a history of cancer. The incidence rate ratio (IRR) for all comorbidities was highest for osteonecrosis (IRR, 8.3), followed by osteoporosis (IRR, 5.75), and joint replacement (IRR, 3.89). The use of methotrexate (IRR, 21.6 for any dose) and corticosteroids (IRR, 5.4 for any dose) was significantly associated with osteonecrosis.[65]
Osteochondroma
- Osteochondromas are benign bony protrusions that can be spontaneous or associated with radiation therapy. They generally occur as a single lesion. However, multiple lesions may develop in the context of hereditary multiple osteochondromatosis.[66]
- Growth hormone therapy may influence the onset and pace of growth of osteochondromas.[14,67]
- Because malignant degeneration of these lesions is exceptionally rare, clinical rather than radiological follow-up is most appropriate.[68]
- Surgical resection is only necessary when the lesion interferes with joint alignment and movement.[69]
Evidence (risk of osteochondroma):
- Approximately 5% of children undergoing myeloablative HSCT will develop osteochondroma, which most commonly presents in the metaphyseal regions of long bones.[66,70]
- A large Italian study reported a 6.1% cumulative risk of developing osteochondroma at 15 years posttransplant, with increased risk associated with younger age at transplant (≤3 years) and use of TBI.[71]
- Osteochondromas have been reported in patients with neuroblastoma who received local radiation therapy, anti-GD2 monoclonal antibody therapy, and isotretinoin.[72]
- Osteochondromas occurred at a median of 8.2 years from diagnosis, and the cumulative incidence rate was 4.9% at 10 years from diagnosis among 362 patients younger than 10 years.
- In this series, most of the osteochondromas were unrelated to radiation and had features characteristic of benign developmental osteochondroma.
- The pathogenic role for chemotherapy, anti-GD2 monoclonal antibody therapy, or isotretinoin in the development of osteochondroma remains speculative.
Amputation and Limb-Sparing Surgery
Amputation and limb-sparing surgery prevent local recurrence of bone tumors by removal of all gross and microscopic disease. If optimally executed, both procedures accomplish an en bloc excision of tumor with a margin of normal uninvolved tissue.
- The type of surgical procedure, the primary tumor site, and the age of the patient affect the risk of postsurgical complications.[73]
- Complications in survivors treated with amputation include prosthetic fit problems, chronic pain in the residual limb, phantom limb pain, and bone overgrowth.[73,74]
- While limb-sparing surgeries may offer a more aesthetically pleasing outcome, complications have been reported more frequently in survivors who underwent these procedures than in those treated with amputation.[73,75]
- Complications after limb-sparing surgery include nonunion, pathological fracture, aseptic loosening, limb-length discrepancy, endoprosthetic fracture, and limited joint range of motion.[73,75]
- Occasionally, refractory complications develop after limb-sparing surgery and require amputation.[76,77]
A number of studies have compared functional outcomes after amputation and limb-sparing surgery, but results have been limited by inconsistent methods of functional assessment and small cohort sizes.
- Overall, data suggest that limb-sparing surgery results in better function than amputation, but differences are relatively modest.[73,77,78]
- Similarly, long-term quality-of-life outcomes among survivors undergoing amputation and limb-sparing procedures have not differed substantially.[76]
- A longitudinal analysis of health status among extremity sarcoma survivors in the CCSS indicates an association between lower extremity amputation and increasing activity limitations with age, and an association between upper extremity amputation and lower educational attainment.[79]
CCSS investigators evaluated risk factors for and outcomes of late amputation in survivors treated for lower extremity sarcomas.[80]
- The cumulative incidence of amputation at 25 years after cancer diagnosis and primary limb-sparing surgery was 18% and did not differ significantly among survivors diagnosed in the 1970s (27%) versus the 1980s (19%) and 1990s (15%).
- Factors associated with an increased risk of late amputation included male sex (hazard ratio [HR], 2.2; 95% CI, 1.07–3.82) and history of prosthetic joint reconstruction (HR, 2.58; 95% CI, 1.37–4.84).
- Survivors treated with primary amputation and those experiencing late amputation after limb-sparing surgery were more likely to report impaired physical functioning (RR, 1.31; 95% CI, 1.04–1.72 and RR, 2.46; 95% CI, 1.66–3.63, respectively) and worse bodily pain scores (RR, 1.50; 95% CI, 1.10–2.06 and RR, 2.33; 95% CI, 1.27–4.26, respectively) than those without amputation after limb-sparing surgery.
The incidence of late major surgical interventions among childhood cancer survivors was examined through data from the CCSS. In the report, survivors of Ewing sarcoma and osteosarcoma had the highest cumulative burden of late, major surgical interventions among all solid tumor survivors (mean cumulative counts of late surgical interventions was 322.9 per 100 survivors of Ewing sarcoma and 269.6 per 100 survivors of osteosarcoma). A large component of this burden is related to the increased rate of additional late musculoskeletal surgeries, such as arthroplasty, amputation, prosthetic revision caused by an infection, device failure, or associated fractures. Locoregional surgery or radiation therapy cancer treatments were associated with undergoing late surgical intervention in the same body region or organ system.[81]
Joint Contractures
HSCT with any history of chronic GVHD is associated with joint contractures.[82,83]
Table 15 summarizes bone and joint late effects and the related health screenings.
Table 15. Bone and Joint Late Effectsa
Predisposing Therapy |
Musculoskeletal Effects |
Health Screening |
CT = computed tomography; DXA = dual-energy x-ray absorptiometry; GVHD = graft-versus-host disease; HSCT = hematopoietic stem cell transplant. |
a Adapted from theChildren's Oncology Group Long-Term Follow-Up Guidelines for Survivors of Childhood, Adolescent, and Young Adult Cancers. |
Radiation exposing musculoskeletal system |
Hypoplasia; fibrosis; reduced/uneven growth (scoliosis, kyphosis); limb length discrepancy |
Examination: bones and soft tissues in radiation fields |
Radiation exposing head and neck |
Craniofacial abnormalities |
History: psychosocial assessment, with attention to educational and/or vocational progress, depression, anxiety, posttraumatic stress, social withdrawal |
Head and neck examination |
Radiation exposing musculoskeletal system |
Radiation-induced fracture |
Examination of affected bone |
Methotrexate; corticosteroids (dexamethasone, prednisone); radiation exposing skeletal structures; HSCT |
Reduced bone mineral density |
Bone mineral density test (DXA or quantitative CT) |
Corticosteroids (dexamethasone, prednisone) |
Osteonecrosis |
History: joint pain, swelling, immobility, limited range of motion |
Musculoskeletal examination |
Radiation with exposure to oral cavity |
Osteoradionecrosis |
History/oral examination: impaired or delayed healing after dental work, persistent jaw pain or swelling, trismus |
Amputation |
Amputation-related complications (impaired cosmesis, functional/activity limitations, residual limb integrity, chronic pain, increased energy expenditure) |
History: pain, functional/activity limitations |
Examination: residual limb integrity |
Prosthetic evaluation |
Limb-sparing surgery |
Limb-sparing surgical complications (functional/activity limitations, fibrosis, contractures, chronic infection, chronic pain, limb length discrepancy, increased energy expenditure, prosthetic malfunction [loosening, nonunion, fracture]) |
History: pain, functional/activity limitations |
Examination: residual limb integrity |
Radiograph of affected limb |
Orthopedic evaluation |
HSCT with any history of chronic GVHD |
Joint contracture |
Musculoskeletal examination |
For musculoskeletal system late effects information, including risk factors, evaluation, and health counseling, see the Children's Oncology Group Long-Term Follow-Up Guidelines for Survivors of Childhood, Adolescent, and Young Adult Cancers.
References:
-
Estilo CL, Huryn JM, Kraus DH, et al.: Effects of therapy on dentofacial development in long-term survivors of head and neck rhabdomyosarcoma: the memorial sloan-kettering cancer center experience. J Pediatr Hematol Oncol 25 (3): 215-22, 2003.
-
Gevorgyan A, La Scala GC, Neligan PC, et al.: Radiation-induced craniofacial bone growth disturbances. J Craniofac Surg 18 (5): 1001-7, 2007.
-
Karsila-Tenovuo S, Jahnukainen K, Peltomäki T, et al.: Disturbances in craniofacial morphology in children treated for solid tumors. Oral Oncol 37 (7): 586-92, 2001.
-
Choi SY, Kim MS, Yoo S, et al.: Long term follow-up results of external beam radiotherapy as primary treatment for retinoblastoma. J Korean Med Sci 25 (4): 546-51, 2010.
-
Shildkrot Y, Kirzhner M, Haik BG, et al.: The effect of cancer therapies on pediatric anophthalmic sockets. Ophthalmology 118 (12): 2480-6, 2011.
-
Brownstein CM, Mertens AC, Mitby PA, et al.: Factors that affect final height and change in height standard deviation scores in survivors of childhood cancer treated with growth hormone: a report from the childhood cancer survivor study. J Clin Endocrinol Metab 89 (9): 4422-7, 2004.
-
Nandagopal R, Laverdière C, Mulrooney D, et al.: Endocrine late effects of childhood cancer therapy: a report from the Children's Oncology Group. Horm Res 69 (2): 65-74, 2008.
-
Sklar CA, Constine LS: Chronic neuroendocrinological sequelae of radiation therapy. Int J Radiat Oncol Biol Phys 31 (5): 1113-21, 1995.
-
Packer RJ, Boyett JM, Janss AJ, et al.: Growth hormone replacement therapy in children with medulloblastoma: use and effect on tumor control. J Clin Oncol 19 (2): 480-7, 2001.
-
Chow EJ, Friedman DL, Yasui Y, et al.: Decreased adult height in survivors of childhood acute lymphoblastic leukemia: a report from the Childhood Cancer Survivor Study. J Pediatr 150 (4): 370-5, 375.e1, 2007.
-
Bongers ME, Francken AB, Rouwé C, et al.: Reduction of adult height in childhood acute lymphoblastic leukemia survivors after prophylactic cranial irradiation. Pediatr Blood Cancer 45 (2): 139-43, 2005.
-
Leung W, Ahn H, Rose SR, et al.: A prospective cohort study of late sequelae of pediatric allogeneic hematopoietic stem cell transplantation. Medicine (Baltimore) 86 (4): 215-24, 2007.
-
Sanders JE: Growth and development after hematopoietic cell transplant in children. Bone Marrow Transplant 41 (2): 223-7, 2008.
-
Sanders JE, Guthrie KA, Hoffmeister PA, et al.: Final adult height of patients who received hematopoietic cell transplantation in childhood. Blood 105 (3): 1348-54, 2005.
-
Shalitin S, Laur E, Lebenthal Y, et al.: Endocrine complications and components of the metabolic syndrome in survivors of childhood malignant non-brain solid tumors. Horm Res Paediatr 81 (1): 32-42, 2014.
-
Silber JH, Littman PS, Meadows AT: Stature loss following skeletal irradiation for childhood cancer. J Clin Oncol 8 (2): 304-12, 1990.
-
Hartley KA, Li C, Laningham FH, et al.: Vertebral body growth after craniospinal irradiation. Int J Radiat Oncol Biol Phys 70 (5): 1343-9, 2008.
-
Paulino AC, Nguyen TX, Mai WY: An analysis of primary site control and late effects according to local control modality in non-metastatic Ewing sarcoma. Pediatr Blood Cancer 48 (4): 423-9, 2007.
-
Oshiro Y, Mizumoto M, Pan H, et al.: Spinal changes after craniospinal irradiation in pediatric patients. Pediatr Blood Cancer 67 (12): e28728, 2020.
-
Hogeboom CJ, Grosser SC, Guthrie KA, et al.: Stature loss following treatment for Wilms tumor. Med Pediatr Oncol 36 (2): 295-304, 2001.
-
Paulino AC, Wen BC, Brown CK, et al.: Late effects in children treated with radiation therapy for Wilms' tumor. Int J Radiat Oncol Biol Phys 46 (5): 1239-46, 2000.
-
Fiscaletti M, Samoilenko M, Dubois J, et al.: Predictors of Vertebral Deformity in Long-Term Survivors of Childhood Acute Lymphoblastic Leukemia: The PETALE Study. J Clin Endocrinol Metab 106 (2): 512-525, 2021.
-
Wilson CL, Dilley K, Ness KK, et al.: Fractures among long-term survivors of childhood cancer: a report from the Childhood Cancer Survivor Study. Cancer 118 (23): 5920-8, 2012.
-
Davies JH, Evans BA, Jenney ME, et al.: Skeletal morbidity in childhood acute lymphoblastic leukaemia. Clin Endocrinol (Oxf) 63 (1): 1-9, 2005.
-
Wilson CL, Chemaitilly W, Jones KE, et al.: Modifiable Factors Associated With Aging Phenotypes Among Adult Survivors of Childhood Acute Lymphoblastic Leukemia. J Clin Oncol 34 (21): 2509-15, 2016.
-
van Atteveld JE, de Winter DTC, Pluimakers VG, et al.: Risk and determinants of low and very low bone mineral density and fractures in a national cohort of Dutch adult childhood cancer survivors (DCCSS-LATER): a cross-sectional study. Lancet Diabetes Endocrinol 11 (1): 21-32, 2023.
-
van der Sluis IM, van den Heuvel-Eibrink MM, Hählen K, et al.: Altered bone mineral density and body composition, and increased fracture risk in childhood acute lymphoblastic leukemia. J Pediatr 141 (2): 204-10, 2002.
-
Arikoski P, Komulainen J, Riikonen P, et al.: Reduced bone density at completion of chemotherapy for a malignancy. Arch Dis Child 80 (2): 143-8, 1999.
-
Brennan BM, Mughal Z, Roberts SA, et al.: Bone mineral density in childhood survivors of acute lymphoblastic leukemia treated without cranial irradiation. J Clin Endocrinol Metab 90 (2): 689-94, 2005.
-
Warner JT, Evans WD, Webb DK, et al.: Relative osteopenia after treatment for acute lymphoblastic leukemia. Pediatr Res 45 (4 Pt 1): 544-51, 1999.
-
Mandel K, Atkinson S, Barr RD, et al.: Skeletal morbidity in childhood acute lymphoblastic leukemia. J Clin Oncol 22 (7): 1215-21, 2004.
-
Gurney JG, Kaste SC, Liu W, et al.: Bone mineral density among long-term survivors of childhood acute lymphoblastic leukemia: results from the St. Jude Lifetime Cohort Study. Pediatr Blood Cancer 61 (7): 1270-6, 2014.
-
den Hoed MA, Klap BC, te Winkel ML, et al.: Bone mineral density after childhood cancer in 346 long-term adult survivors of childhood cancer. Osteoporos Int 26 (2): 521-9, 2015.
-
den Hoed MA, Pluijm SM, te Winkel ML, et al.: Aggravated bone density decline following symptomatic osteonecrosis in children with acute lymphoblastic leukemia. Haematologica 100 (12): 1564-70, 2015.
-
Kvammen JA, Stensvold E, Godang K, et al.: Bone mineral density and nutrition in long-term survivors of childhood brain tumors. Clin Nutr ESPEN 50: 162-169, 2022.
-
Le Meignen M, Auquier P, Barlogis V, et al.: Bone mineral density in adult survivors of childhood acute leukemia: impact of hematopoietic stem cell transplantation and other treatment modalities. Blood 118 (6): 1481-9, 2011.
-
Kodama M, Komura H, Shimizu S, et al.: Efficacy of hormone therapy for osteoporosis in adolescent girls after hematopoietic stem cell transplantation: a longitudinal study. Fertil Steril 95 (2): 731-5, 2011.
-
Bhandari R, Teh JB, Herrera C, et al.: Prevalence and risk factors for vitamin D deficiency in long-term childhood cancer survivors. Pediatr Blood Cancer 68 (7): e29048, 2021.
-
Paulino AC: Late effects of radiotherapy for pediatric extremity sarcomas. Int J Radiat Oncol Biol Phys 60 (1): 265-74, 2004.
-
van Atteveld JE, Pluijm SMF, Ness KK, et al.: Prediction of Low and Very Low Bone Mineral Density Among Adult Survivors of Childhood Cancer. J Clin Oncol 37 (25): 2217-2225, 2019.
-
Mattano LA, Devidas M, Nachman JB, et al.: Effect of alternate-week versus continuous dexamethasone scheduling on the risk of osteonecrosis in paediatric patients with acute lymphoblastic leukaemia: results from the CCG-1961 randomised cohort trial. Lancet Oncol 13 (9): 906-15, 2012.
-
Bürger B, Beier R, Zimmermann M, et al.: Osteonecrosis: a treatment related toxicity in childhood acute lymphoblastic leukemia (ALL)--experiences from trial ALL-BFM 95. Pediatr Blood Cancer 44 (3): 220-5, 2005.
-
Karimova EJ, Rai SN, Howard SC, et al.: Femoral head osteonecrosis in pediatric and young adult patients with leukemia or lymphoma. J Clin Oncol 25 (12): 1525-31, 2007.
-
Campbell S, Sun CL, Kurian S, et al.: Predictors of avascular necrosis of bone in long-term survivors of hematopoietic cell transplantation. Cancer 115 (18): 4127-35, 2009.
-
Kawedia JD, Kaste SC, Pei D, et al.: Pharmacokinetic, pharmacodynamic, and pharmacogenetic determinants of osteonecrosis in children with acute lymphoblastic leukemia. Blood 117 (8): 2340-7; quiz 2556, 2011.
-
Girard P, Auquier P, Barlogis V, et al.: Symptomatic osteonecrosis in childhood leukemia survivors: prevalence, risk factors and impact on quality of life in adulthood. Haematologica 98 (7): 1089-97, 2013.
-
Ali N, Gohar S, Zaky I, et al.: Osteonecrosis in children with acute lymphoblastic leukemia: A report from Children's Cancer Hospital Egypt (CCHE). Pediatr Blood Cancer 66 (1): e27440, 2019.
-
Karimova EJ, Wozniak A, Wu J, et al.: How does osteonecrosis about the knee progress in young patients with leukemia?: a 2- to 7-year study. Clin Orthop Relat Res 468 (9): 2454-9, 2010.
-
Padhye B, Dalla-Pozza L, Little D, et al.: Incidence and outcome of osteonecrosis in children and adolescents after intensive therapy for acute lymphoblastic leukemia (ALL). Cancer Med 5 (5): 960-7, 2016.
-
te Winkel ML, Pieters R, Hop WC, et al.: Prospective study on incidence, risk factors, and long-term outcome of osteonecrosis in pediatric acute lymphoblastic leukemia. J Clin Oncol 29 (31): 4143-50, 2011.
-
DeFeo BM, Kaste SC, Li Z, et al.: Long-Term Functional Outcomes Among Childhood Survivors of Cancer Who Have a History of Osteonecrosis. Phys Ther 100 (3): 509-522, 2020.
-
Hernigou P, Auregan JC, Dubory A, et al.: Ankle osteonecrosis in fifty-one children and adolescent's leukemia survivors: a prospective randomized study on percutaneous mesenchymal stem cells treatment. Int Orthop 45 (9): 2383-2393, 2021.
-
Faraci M, Calevo MG, Lanino E, et al.: Osteonecrosis after allogeneic stem cell transplantation in childhood. A case-control study in Italy. Haematologica 91 (8): 1096-9, 2006.
-
Relling MV, Yang W, Das S, et al.: Pharmacogenetic risk factors for osteonecrosis of the hip among children with leukemia. J Clin Oncol 22 (19): 3930-6, 2004.
-
Vrooman LM, Stevenson KE, Supko JG, et al.: Postinduction dexamethasone and individualized dosing of Escherichia Coli L-asparaginase each improve outcome of children and adolescents with newly diagnosed acute lymphoblastic leukemia: results from a randomized study--Dana-Farber Cancer Institute ALL Consortium Protocol 00-01. J Clin Oncol 31 (9): 1202-10, 2013.
-
Hyakuna N, Shimomura Y, Watanabe A, et al.: Assessment of corticosteroid-induced osteonecrosis in children undergoing chemotherapy for acute lymphoblastic leukemia: a report from the Japanese Childhood Cancer and Leukemia Study Group. J Pediatr Hematol Oncol 36 (1): 22-9, 2014.
-
Badhiwala JH, Nayiager T, Athale UH: The development of thromboembolism may increase the risk of osteonecrosis in children with acute lymphoblastic leukemia. Pediatr Blood Cancer 62 (10): 1851-4, 2015.
-
Li X, Brazauskas R, Wang Z, et al.: Avascular necrosis of bone after allogeneic hematopoietic cell transplantation in children and adolescents. Biol Blood Marrow Transplant 20 (4): 587-92, 2014.
-
Mattano LA, Sather HN, Trigg ME, et al.: Osteonecrosis as a complication of treating acute lymphoblastic leukemia in children: a report from the Children's Cancer Group. J Clin Oncol 18 (18): 3262-72, 2000.
-
Karol SE, Mattano LA, Yang W, et al.: Genetic risk factors for the development of osteonecrosis in children under age 10 treated for acute lymphoblastic leukemia. Blood 127 (5): 558-64, 2016.
-
Finkelstein Y, Blonquist TM, Vijayanathan V, et al.: A thymidylate synthase polymorphism is associated with increased risk for bone toxicity among children treated for acute lymphoblastic leukemia. Pediatr Blood Cancer 64 (7): , 2017.
-
Karol SE, Yang W, Van Driest SL, et al.: Genetics of glucocorticoid-associated osteonecrosis in children with acute lymphoblastic leukemia. Blood 126 (15): 1770-6, 2015.
-
Aricò M, Boccalatte MF, Silvestri D, et al.: Osteonecrosis: An emerging complication of intensive chemotherapy for childhood acute lymphoblastic leukemia. Haematologica 88 (7): 747-53, 2003.
-
Elmantaser M, Stewart G, Young D, et al.: Skeletal morbidity in children receiving chemotherapy for acute lymphoblastic leukaemia. Arch Dis Child 95 (10): 805-9, 2010.
-
Chao C, Bhatia S, Xu L, et al.: Chronic Comorbidities Among Survivors of Adolescent and Young Adult Cancer. J Clin Oncol 38 (27): 3161-3174, 2020.
-
Bovée JV: Multiple osteochondromas. Orphanet J Rare Dis 3: 3, 2008.
-
Bordigoni P, Turello R, Clement L, et al.: Osteochondroma after pediatric hematopoietic stem cell transplantation: report of eight cases. Bone Marrow Transplant 29 (7): 611-4, 2002.
-
Taitz J, Cohn RJ, White L, et al.: Osteochondroma after total body irradiation: an age-related complication. Pediatr Blood Cancer 42 (3): 225-9, 2004.
-
King EA, Hanauer DA, Choi SW, et al.: Osteochondromas after radiation for pediatric malignancies: a role for expanded counseling for skeletal side effects. J Pediatr Orthop 34 (3): 331-5, 2014 Apr-May.
-
Danner-Koptik K, Kletzel M, Dilley KJ: Exostoses as a long-term sequela after pediatric hematopoietic progenitor cell transplantation: potential causes and increase risk of secondary malignancies from Ann & Robert H. Lurie Children's Hospital of Chicago. Biol Blood Marrow Transplant 19 (8): 1267-70, 2013.
-
Faraci M, Bagnasco F, Corti P, et al.: Osteochondroma after hematopoietic stem cell transplantation in childhood. An Italian study on behalf of the AIEOP-HSCT group. Biol Blood Marrow Transplant 15 (10): 1271-6, 2009.
-
Kushner BH, Roberts SS, Friedman DN, et al.: Osteochondroma in long-term survivors of high-risk neuroblastoma. Cancer 121 (12): 2090-6, 2015.
-
Nagarajan R, Neglia JP, Clohisy DR, et al.: Limb salvage and amputation in survivors of pediatric lower-extremity bone tumors: what are the long-term implications? J Clin Oncol 20 (22): 4493-501, 2002.
-
Aulivola B, Hile CN, Hamdan AD, et al.: Major lower extremity amputation: outcome of a modern series. Arch Surg 139 (4): 395-9; discussion 399, 2004.
-
Kaste SC, Neel MN, Rao BN, et al.: Complications of limb-sparing procedures using endoprosthetic replacements about the knee for pediatric skeletal sarcomas. Pediatr Radiol 31 (2): 62-71, 2001.
-
Eiser C, Darlington AS, Stride CB, et al.: Quality of life implications as a consequence of surgery: limb salvage, primary and secondary amputation. Sarcoma 5 (4): 189-95, 2001.
-
Renard AJ, Veth RP, Schreuder HW, et al.: Function and complications after ablative and limb-salvage therapy in lower extremity sarcoma of bone. J Surg Oncol 73 (4): 198-205, 2000.
-
Fernandez-Pineda I, Hudson MM, Pappo AS, et al.: Long-term functional outcomes and quality of life in adult survivors of childhood extremity sarcomas: a report from the St. Jude Lifetime Cohort Study. J Cancer Surviv 11 (1): 1-12, 2017.
-
Marina N, Hudson MM, Jones KE, et al.: Changes in health status among aging survivors of pediatric upper and lower extremity sarcoma: a report from the childhood cancer survivor study. Arch Phys Med Rehabil 94 (6): 1062-73, 2013.
-
Geiger EJ, Liu W, Srivastava DK, et al.: What Are Risk Factors for and Outcomes of Late Amputation After Treatment for Lower Extremity Sarcoma: A Childhood Cancer Survivor Study Report. Clin Orthop Relat Res 481 (3): 526-538, 2023.
-
Dieffenbach BV, Murphy AJ, Liu Q, et al.: Cumulative burden of late, major surgical intervention in survivors of childhood cancer: a report from the Childhood Cancer Survivor Study (CCSS) cohort. Lancet Oncol 24 (6): 691-700, 2023.
-
Beredjiklian PK, Drummond DS, Dormans JP, et al.: Orthopaedic manifestations of chronic graft-versus-host disease. J Pediatr Orthop 18 (5): 572-5, 1998 Sep-Oct.
-
Inamoto Y, Storer BE, Petersdorf EW, et al.: Incidence, risk factors, and outcomes of sclerosis in patients with chronic graft-versus-host disease. Blood 121 (25): 5098-103, 2013.
Late Effects of the Reproductive System
Reproductive outcomes in childhood cancer survivors may be compromised by surgery, radiation therapy, or chemotherapy that negatively affects any component of the hypothalamic-pituitary axis or gonads. Evidence for this outcome in childhood cancer survivors is limited by studies characterized by small sample size, cohort selection and participation bias, cross-sectional assessment, heterogeneity in treatment approach, time since treatment, and method of ascertainment. In particular, the literature is deficient regarding hard outcomes of reproductive potential (e.g., semen analysis in men, primordial follicle count in women) and outcomes after contemporary risk-adapted treatment approaches.[1,2]
The risk of infertility is generally related to the tissues or organs involved by the cancer and the specific type, dose, and combination of cytotoxic therapy.
- In pediatric patients with germ cell tumors, orchiectomy or oophorectomy may reduce germ cell numbers.
- Alkylating agents and similar DNA interstrand cross-linking agents are used in the treatment of pediatric cancers. They are the primary chemotherapeutic agents associated with a high risk of infertility. Factors influencing the risk of gonadal injury in children treated with alkylating agent chemotherapy include the following:
- Cumulative dose.
Earlier studies used the alkylating agent dose to define dose levels associated with the risk of gonadal toxicity within a specific study cohort. Childhood Cancer Survivor Study (CCSS) investigators developed the cyclophosphamide equivalent dose, which is a metric for normalization of the cumulative doses of various alkylating agents that is independent of the study population. The alkylating agent dose and cyclophosphamide equivalent dose perform similarly when used in several models for different survivor outcomes that include treatment exposures, but only the cyclophosphamide equivalent dose permits comparison across variably treated cohorts. Investigations that evaluate risk factors for gonadal toxicity vary in the use of cumulative doses based on individual alkylating agents, the alkylating agent dose, and the cyclophosphamide equivalent dose.[3]
- The specific alkylating agent.
- The length of treatment.
- Age at treatment.
- Sex.
- The risk of radiation injury to the hypothalamic-pituitary axis or gonads is related to the treatment volume, total dose, fractionation schedule, and age at treatment.
The St. Jude Children's Research Hospital reported the cumulative risk of hypogonadism and infertility in a cohort of 156 pediatric patients with medulloblastomas who were treated with surgery, risk-adapted craniospinal irradiation, and dose-intensive chemotherapy in the SJMB03 study (2003–2013).[4]
- For the entire cohort, the 5-year cumulative incidence of hypogonadism was 33.9%, and the 10-year cumulative incidence was 45.1%.
- The 10-year cumulative incidence of hypogonadism was 82.4% for women and 18% for men (P < .0001).
- The incidence was 62.9% for patients who had achieved puberty at the time of radiation therapy and 40.1% for those who had not achieved puberty (P = .0068).
- The incidence was 80.6% for those who received a high gonadal radiation dose (>2 Gy), 67.7% for those who received an intermediate gonadal radiation dose (1–2 Gy), and 31.9% for those who received a low gonadal radiation dose (<1 Gy) (P < .0001).
- Hypogonadism was associated with sex, puberty status, gonadal radiation dose exposure, interval from treatment to puberty, and vincristine dose.
- The cumulative incidence of infertility at 10 years was 55.4% for women and 23.5% for men (n = 33; P = .0389).
In addition to anticancer therapy, age at treatment, and sex, it is likely that genetic factors influence the risk of permanent infertility. Pediatric cancer treatment protocols often prescribe combined-modality therapy; thus, the additive effects of gonadotoxic exposures may need to be considered in assessing reproductive potential. Detailed information about the specific cancer treatment modalities, including specific surgical procedures, the type and cumulative doses of chemotherapeutic agents, and radiation treatment volumes and doses, are needed to estimate risks for gonadal dysfunction and infertility.
The treatment-indicated risk of infertility does not simply translate into adult fertility status. This is particularly important for patients who were unable to participate in sperm/oocyte preservation because of their young age when diagnosed and treated for cancer.
- In a study of survivors from a St. Jude Life (SJLIFE) cohort (N = 1,067), most adult survivors of childhood cancer (61.9%) considered themselves at risk of infertility, which was significantly associated with sociodemographic factors (older age, White ethnicity, being married/partnered, higher education), gonadotoxic treatment, fertility concerns, previous unsuccessful attempts to conceive, and sexual dysfunction (all P < .05).[5]
- Risk perceptions were often discordant from laboratory-evaluated gonadal functioning. Laboratory-evaluated impaired gonadal function was found in 24% of female survivors and 56% of male survivors, but concordance with survivors' risk perceptions was low. Most survivors with discordant perceptions overestimated risk (20% of male and 44% of female survivors).
- Survivors who are at risk—such as those who show initial signs of fertility problems and/or those who are experiencing fertility-related uncertainties, worries, or distress—should be offered fertility testing.
Testis
Cancer treatments that may impair testicular and reproductive function include the following:
- Surgery (orchiectomy, retroperitoneal lymph node dissection, extensive pelvic dissection).
- Radiation therapy (exposing the hypothalamic-pituitary axis or testes).
- Chemotherapy (alkylating agents and similar DNA interstrand cross-linking agents such as procarbazine).
- Hematopoietic stem cell transplant (HSCT).
Surgery affecting testicular function
- Patients who undergo unilateral orchiectomy for testicular torsion may have subnormal sperm counts at long-term follow-up.[6,7]
- Retrograde ejaculation is a frequent complication of bilateral retroperitoneal lymph node dissection performed on males with testicular neoplasms.[8,9] Long-term outcomes after minimally invasive retroperitoneal lymph node dissection are not available.[10]
- Erectile dysfunction may occur after extensive pelvic dissections to remove a rhabdomyosarcoma of the prostate.[11,12]
Radiation affecting testicular function
Among men who were treated for childhood cancer, gonadal injury may have occurred if radiation treatment fields included the pelvis, gonads, or total body.
- The germinal epithelium is more sensitive to radiation injury than the androgen-producing Leydig cells.
- A decrease in sperm counts can be seen 3 to 6 weeks after such irradiation. Depending on the dosage, recovery may take 1 to more than 3 years.[13]
- The germinal epithelium is damaged by much lower dosages (<1 Gy) of radiation than are Leydig cells (20–30 Gy).[13]
- Irreversible germ cell failure may occur with fractionated radiation doses of greater than 2 Gy to 4 Gy.[13]
- Administration of higher radiation doses, such as 24 Gy, which was used for the treatment of testicular relapse of acute lymphoblastic leukemia (ALL), results in both germ cell failure and Leydig cell dysfunction.[14]
Radiation injury to Leydig cells is related to the dose delivered and age at treatment.
- Testosterone production may be normal in prepubertal boys treated with less than 12 Gy fractionated testicular irradiation, but elevated plasma concentrations of luteinizing hormone observed in this group suggest subclinical injury.[15]
- Leydig cell failure typically results when prepubertal boys are treated with more than 20 Gy of radiation to the testes. Androgen therapy is required for masculinization.[15]
- Leydig cell function is usually preserved in sexually mature male patients if radiation doses do not exceed 30 Gy.[15]
- Although available data suggest that Leydig cells are more vulnerable when exposed to radiation before puberty, confounding factors, such as the age at testing and the effects of both orchiectomy and chemotherapy, limit the reliability of this observation.[15]
Chemotherapy affecting testicular function
Cumulative alkylating agent (e.g., cyclophosphamide, mechlorethamine, dacarbazine) dose is an important factor in estimating the risk of testicular germ cell injury. However, limited data are available that correlate results of semen analyses in clinically well-characterized cohorts.[16]
- In general, Leydig cell function is preserved, but germ cell failure is common in men treated with high cumulative doses of cyclophosphamide (7,500 mg/m2 or more) and more than 3 months of combination alkylating agent therapy.
- Most studies suggest that prepubertal males are not at lower risk of chemotherapy-induced testicular damage than are postpubertal patients.[17,18,19,20]
Studies of testicular germ cell injury, as evidenced by oligospermia or azoospermia, after alkylating agent administration with or without radiation therapy have reported the following:
-
Cyclophosphamide:
- Male survivors of non-Hodgkin lymphoma who received a cumulative cyclophosphamide dose of greater than 9.5 g/m2 and underwent pelvic radiation therapy were at increased risk of failure to recover spermatogenesis.[21]
- In survivors of Ewing sarcoma and soft tissue sarcoma, treatment with a cumulative cyclophosphamide dose of greater than 7.5 g/m2 was correlated with persistent oligospermia or azoospermia.[22]
- Cyclophosphamide doses exceeding 7.5 g/m2 and ifosfamide doses exceeding 60 g/m2 produced oligospermia or azoospermia in most exposed individuals.[23,24,25]
- A small cohort study reported normal semen quality in adult long-term survivors of childhood ALL treated with 0 to 10 g/m2 of cyclophosphamide and cranial irradiation, whereas no spermatozoa were detected in semen samples from survivors treated with more than 20 g/m2 of cyclophosphamide.[26]
- Treatment with a cyclophosphamide equivalent dose of less than 4 g/m2 results in infrequent azoospermia or oligospermia. Approximately 89% of 31 men treated were normospermic.[27]
- Spermatogenesis was present in 67% of 15 men who received 200 mg/kg of cyclophosphamide before undergoing HSCT for aplastic anemia.[28]
-
Dacarbazine:
- The combination of doxorubicin, bleomycin, vinblastine, and dacarbazine (ABVD) frequently produced oligospermia or azoospermia in adults during the course of treatment. However, recovery of spermatogenesis occurred after treatment was completed, which is in contrast to the experience reported after treatment with mechlorethamine, vincristine, procarbazine, and prednisone (MOPP).[29]
-
Alkylating agent plus procarbazine:
- Most studies suggest that combination chemotherapy with an alkylating agent and procarbazine causes severe damage to the testicular germinal epithelium that is irreversible at high cumulative doses.[17,30,31,32,33]
- Azoospermia occurred less frequently in adults after treatment with two, rather than six, cycles of MOPP.[34]
- Elevation of the basal follicle-stimulating hormone (FSH) level, which may reflect impaired spermatogenesis, was less frequent among patients who received two courses of vincristine, procarbazine, prednisone, and doxorubicin (OPPA) than among those who received two courses of OPPA in combination with two or more courses of cyclophosphamide, vincristine, procarbazine and prednisone (COPP).[35]
-
Alkylating agents plus low-dose cranial radiation:
- In a cross-sectional study that included male adult survivors of pediatric ALL who had received alkylating agent chemotherapy with or without cranial radiation, St. Jude Children's Research Hospital investigators demonstrated that cranial radiation at doses lower than 26 Gy has no demonstrable independent effect on spermatogenesis.[36]
Testicular function after hematopoietic stem cell transplant (HSCT)
The risk of gonadal dysfunction and infertility related to conditioning with total-body irradiation (TBI), high-dose alkylating agent chemotherapy, or both is substantial.[37] Because patients with relapsed or refractory cancer often undergo HSCT, previous treatment with alkylating agent chemotherapy or hypothalamic-pituitary axis or gonadal radiation therapy may confer additional risks.
- A retrospective longitudinal analysis of data obtained over a 30-year period from a tertiary care HSCT unit included 130 males who were younger than 18 years when they underwent a transplant and who survived 2 years or longer after HSCT. Fifty-six percent of the patients were prepubertal, while 44% were peri- or postpubertal when undergoing an HSCT.[38]
- Patients who were prepubertal before undergoing HSCT had a reduced risk of developing hypogonadism after HSCT (OR, 0.15), despite overall smaller testicular volume achieved in adulthood.
- Chemotherapy-only regimens were associated with statistically larger testicular volume (P < .001), higher testosterone levels (P = .008), and lower gonadotropin levels (P < .001) than TBI.
- Evidence of progressively declining Leydig cell function over time was seen by a downward trend in testosterone levels after the achievement of full pubertal attainment, despite a compensatory increase in LH levels.
- TBI and busulfan exposures were associated with the lowest probability of gonadal event–free courses (P < .0001).
- A French L.E.A. long-term follow-up study evaluated testosterone deficiency in male childhood leukemia survivors after treatment with HSCT or testicular irradiation.[37]
- Among 178 patients treated with myeloablative TBI, 55.6% had total testosterone deficiency, 15.7% had partial deficiency, and 28.7% were normal. A testicular boost of 4 to 6 Gy of radiation therapy and a younger age at HSCT significantly increased the risk.
- Among 53 patients who received a busulfan-containing myeloablative conditioning regimen, 28.3% had total deficiency, 15.1% had partial deficiency, 56.6% were normal. For patients who received this regimen and additional testicular radiation, 0% were normal, compared with 62.5% of patients who did not receive additional testicular radiation.
- Treatment with 24 Gy of testicular radiation without HSCT induced total or partial deficiency in 71.4% and 28.6% of patients, respectively (n = 21).
- Age at treatment also influences the risk of gonadal injury. Young boys and adolescents treated with high-dose cyclophosphamide (200 mg/kg) will generally maintain Leydig cell function and testosterone production, but germ cell failure is common.[39]
- After TBI conditioning, most male patients retain their ability to produce testosterone but will experience germ cell failure.[39]
- Limited data suggest that a greater proportion of boys will retain germinal function or recovery of spermatogenesis (based on pubertal progress and gonadotropin levels) after reduced-intensity conditioning with fludarabine/melphalan than will those treated with myeloablative conditioning with busulfan/cyclophosphamide.[40]
Recovery of germ cell function
Recovery of germ cell function after cytotoxic chemotherapy and radiation therapy is possible. However, evidence based on hard outcomes like sperm count is limited. Most studies use hormonal biomarkers like inhibin B and FSH levels to estimate the presence of spermatogenesis. However, limitations in the specificity and positive predictive value of these tests have been reported.[41,42] Male survivors should be advised that semen analysis is the most accurate assessment of adequacy of spermatogenesis.
Leydig cell function in long-term survivors of childhood cancer
Leydig cell function in childhood cancer survivors has not been well studied. SJLIFE study investigators evaluated the prevalence of and risk factors for Leydig cell failure and Leydig cell dysfunction in 1,516 men (median age, 30.8 years; median time from diagnosis, 22 years).[43]
- The prevalence of Leydig cell failure (defined as testosterone <250 ng/dL and luteinizing hormone >9.85 IU/L) was 6.9%.
- The prevalence of Leydig cell dysfunction (defined as testosterone ≥250 ng/dL and luteinizing hormone >9.85 IU/L) was 14.7%.
- Independent risk factors for Leydig cell failure included attained age of 26 years or older at assessment, testicular radiation therapy at any dose, and alkylating agents at cyclophosphamide equivalent doses of 4,000 mg/m2 or higher.
- Unilateral orchiectomy and similar risk factors for Leydig cell failure were associated with Leydig cell dysfunction.
- The risk of Leydig cell failure and dysfunction increased with older age, higher radiation doses, and increased alkylating agent exposure.
- Adverse outcomes significantly associated with Leydig cell failure (but not Leydig cell dysfunction) included abdominal obesity, diabetes mellitus, erectile dysfunction, muscle weakness, and all-cause mortality.
Ovary
Cancer treatments that may impair ovarian function/reserve include the following:
- Surgery (oophorectomy).
- Radiation therapy (exposing the hypothalamic-pituitary axis or ovaries).
- Chemotherapy (alkylating agents, similar DNA interstrand cross-linking agents like procarbazine).
- HSCT.
Surgery affecting ovarian function
The main challenge for the pediatric surgeon in the management of ovarian tumors is finding the right balance between optimal tumor resection and maximal fertility preservation. Oophorectomy performed for the management of germ cell tumors may reduce ovarian reserve. Contemporary treatments use fertility-sparing surgical procedures combined with systemic chemotherapy to reduce this risk.[44]
Radiation affecting ovarian function
In women treated for childhood cancer, primary gonadal injury may have occurred if treatment fields involved the lumbosacral spine, abdomen, pelvis, or total body. The frequency of ovarian failure after abdominal radiation therapy is related to both the age of the patient at the time of irradiation and the radiation therapy dose received by the ovaries. The ovaries of younger individuals are more resistant to radiation damage than those of older women because of their greater complement of primordial follicles.
Whole-abdomen irradiation at doses of 20 Gy or higher is associated with the greatest risk of ovarian dysfunction. Seventy-one percent of patients in one series failed to enter puberty, and 26% of women experienced premature menopause after receiving whole-abdominal radiation therapy doses of 20 Gy to 30 Gy.[45] Other studies reported similar results in women treated with whole-abdomen irradiation [46] or craniospinal irradiation [47,48] during childhood.
Chemotherapy affecting ovarian function
Ovarian function may be impaired after treatment with combination chemotherapy that includes an alkylating agent and procarbazine. In general, girls maintain gonadal function at higher cumulative alkylating agent doses than do boys. Most female childhood cancer survivors who are treated with risk-adapted combination chemotherapy retain or recover ovarian function. However, the risk of acute ovarian failure and premature menopause is substantial if treatment includes combined-modality therapy with alkylating agent chemotherapy and abdominal or pelvic radiation therapy or dose-intensive alkylating agents for myeloablative conditioning before HSCT.[49,50,51,52,53,54]
Premature ovarian failure
Premature ovarian failure is well documented in childhood cancer survivors, especially in women treated with both an alkylating agent and abdominal radiation therapy.[49,53,54,55,56]
Studies have associated the following factors with an increased rate of premature ovarian insufficiency (acute ovarian failure and premature menopause):
- Age at the time of treatment and attained age.
- Increasing doses of abdominal-pelvic radiation therapy.
- Exposure to alkylating agents and/or procarbazine.
- Oophorectomy.
The presence of apparently normal ovarian function at the completion of chemotherapy should not be interpreted as evidence that no ovarian injury has occurred.
Evidence (excess risk of premature ovarian insufficiency after chemotherapy and radiation):
- A CCSS study of 2,008 survivors of unilateral nonsyndromic Wilms tumor found the following:[57]
- The 35-year cumulative incidence of premature ovarian insufficiency was 7.3% (95% confidence interval [CI], 5.0–9.7).
- Survivors of Wilms tumor who were treated with more intense therapy (including radiation therapy) experienced greater rates of premature ovarian insufficiency, compared with survivors who were treated with vincristine and dactinomycin alone.
- The menopausal status of 2,930 survivors (median age, 35 years) participating in the CCSS was compared with that of 1,399 siblings. Nonsurgical premature menopause was defined as sustained menses cessation occurring for more than 6 months, beginning 5 years after the cancer diagnosis but before age 40 years, that was not caused by pregnancy, surgery, or medications.[53]
- The prevalence of nonsurgical premature menopause was 9.1% at age 40 years in these survivors.
- In multivariable analyses, significant independent risk factors for the development of nonsurgical premature menopause were the following:
- Exposure to a dose of procarbazine greater than 4,000 mg/m2 (odds ratio [OR], 8.96; 95% CI, 5.02–16.00; P < .0001).
- Exposure to any dose of radiation therapy to the ovaries (OR, 2.73 [95% CI, 1.33–5.61; P = .0062] for a dose of less than 5 Gy and OR, 8.02 [95% CI, 2.81–22.85; P < .0001] for a dose of greater than 5 Gy).
- Receipt of an HSCT (OR, 6.35; 95% CI, 1.19–33.93; P = .0307).
- A cyclophosphamide equivalent dose of 6,000 mg/m2 or higher that included procarbazine was significant in the univariate analysis, but it did not achieve significance in the multivariable analysis.
- For survivors who received more than 4,000 mg/m2 of procarbazine, the prevalence of nonsurgical premature menopause at age 40 years was 39.7%, compared with 4.2% among those who did not receive any procarbazine (P < .0001).
- Radiation exposure to the ovaries of greater than 5 Gy resulted in a prevalence of nonsurgical premature menopause at age 40 years of 24.1%, compared with a prevalence of 3.0% in those who did not receive radiation (P < .0001).
- Cyclophosphamide exposure (at any dose), unilateral oophorectomy, smoking status, and body mass index (BMI) were not found to be significant for the risk of nonsurgical premature menopause.
- Compared with survivors who did not develop nonsurgical premature menopause, those who developed nonsurgical premature menopause were less likely to ever be pregnant or to have a live birth between the ages of 31 and 40 years. There was no difference in the pregnancy and live birth rates before the age of 30 years for survivors who ultimately developed nonsurgical premature menopause and those who do not.
- A French cohort study of 1,109 female survivors of childhood solid cancer identified the risk factors for nonsurgical menopause, which included treatment with alkylating agents, radiation exposing the ovaries, and oophorectomy.[56]
- Women treated with alkylating agents after the onset of puberty, either alone (relative risk [RR], 9.0; 95% CI, 2.7–28.0; P = .0003) or associated with a low dose of radiation to the ovaries (RR, 29; 95% CI, 8–108; P < .0001), had the highest risk ratio for nonsurgical menopause.
- The overall rate of nonsurgical menopause by age 40 years was only 2.1% and substantially lower than the CCSS and European Organization for Research and Treatment of Cancer cohort studies that include survivors of hematological malignancies.
- Unilateral oophorectomy was associated with a 7-year-earlier age at menopause.
- European survivors of Hodgkin lymphoma treated between the ages 15 years and 40 years and who were not receiving hormonal contraceptives were surveyed for the occurrence of premature ovarian failure.[55]
- In 460 women, premature ovarian failure was mainly influenced by alkylating chemotherapy use with a linear dose relationship between alkylating chemotherapy and premature ovarian failure occurrence.
- Premature ovarian failure risk increased by 23% per year of age at treatment. In women treated without alkylating chemotherapy before age 32 years, cumulative premature ovarian failure risks were 3%, and at age 32 years or older, the same risks were 9%.
- If menstruation returned after treatment, cumulative premature ovarian failure risk was independent of age at treatment.
- Among women who ultimately developed premature ovarian failure, 22% had one or more children after treatment, compared with 41% of women without premature ovarian failure who had one or more children after treatment.
- This report indicates that women with proven fertility after treatment can still face infertility problems at a later stage.
- SJLIFE study investigators evaluated the prevalence of and risk factors for premature ovarian insufficiency in 921 female childhood cancer survivor participants. Premature ovarian insufficiency was clinically assessed and defined by persistent amenorrhea combined with an FSH level of 30 IU/L or higher before age 40 years.[58]
- The prevalence of premature ovarian insufficiency was 10.9% among women who were a median age of 31.7 years at study assessment and a median 24 years from cancer diagnosis.
- Independent risk factors for premature ovarian insufficiency included ovarian radiation therapy at any dose and cyclophosphamide equivalent dose of 8,000 mg/m2 or higher.
- Obesity (BMI of 30 kg/m2 or higher) at assessment was associated with a lower risk of premature ovarian insufficiency (HR, 0.36).
- Survivors with premature ovarian insufficiency had increased odds of low bone mineral density (OR, 5.07) and frailty (OR, 3.5) than did those without premature ovarian insufficiency.
Ovarian function after HSCT
The preservation of ovarian function among women treated with HSCT is related to age at treatment, receipt of pretransplant alkylating agent chemotherapy and abdominal-pelvic radiation therapy, and transplant conditioning regimen.[51,59]
Evidence (excess risk of premature ovarian insufficiency after HSCT):
- A retrospective observational study included 178 women survivors of childhood leukemia from the long-term French follow-up program. The study examined women who were prepubertal when they underwent an HSCT for acute leukemia. Among these survivors, 116 had received TBI and 62 had received a busulfan-based conditioning protocol. The median follow-up from HSCT was 18 years.[60]
- Among 178 women, 106 (60%) needed pubertal induction (with hormone substitution treatment), whereas 72 (40%) had spontaneous menarche.
- After spontaneous menarche, 33 patients (46%) developed premature ovarian insufficiency, mostly within 5 years of HSCT.
- Risk factors for developing premature ovarian insufficiency were older age at the time of HSCT and cryopreservation of ovarian tissue.
- In patients who underwent HSCT before the age of 4.8 years, more than 65% had spontaneous menarche, and almost 50% did not have premature ovarian insufficiency.
- In patients who underwent HSCT after the age of 10.9 years, more than 85% did not have spontaneous menarche and needed induction of puberty (with hormone replacement therapy).
- Girls and young women conditioned with TBI or busulfan-based regimens appear to be at equally high risk of declining ovarian function and premature menopause compared with patients conditioned with cyclophosphamide only.[51] All women who received high-dose (50 mg/kg/day × 4 days) cyclophosphamide before HSCT for aplastic anemia developed amenorrhea after transplant.
- In another series, 36 of 43 women with aplastic anemia conditioned with cyclophosphamide (200 mg/kg) had recovery of normal ovarian function 3 to 42 months after transplant, including all of the 27 patients who were between ages 13 and 25 years at the time of HSCT.[52]
- TBI is especially damaging when given in a single fraction.[51] Most postpubertal women who receive TBI before HSCT develop amenorrhea.
- In one series, recovery of normal ovarian function occurred in only 9 of 144 patients and was highly correlated with age at time of radiation therapy in patients younger than 25 years.[52]
- Among women with leukemia, cranial irradiation before transplant further decreased the possibility of retaining ovarian function.[51]
- Ovarian function may be better preserved (based on pubertal progress and gonadotropin levels) in females undergoing HSCT with reduced-intensity conditioning using fludarabine/melphalan than in those undergoing conditioning with myeloablative busulfan/cyclophosphamide.[40]
Predicting premature ovarian failure
- Using data from the CCSS, investigators developed treatment-informed prediction models to estimate survivors' risks of developing primary ovarian insufficiency. These models provide patient-specific risk across the reproductive age spectrum. Previous models were used to estimate the survivors' risks of developing acute ovarian failure within 5 years of cancer diagnosis.[61] Information from these models can inform discussions surrounding the choice and timing of therapies to preserve fertility. The SJLIFE cohort was used to validate the first age-specific risk prediction models for primary ovarian insufficiency. There were 7,891 female CCSS survivors (922 with primary ovarian insufficiency) included in the development of the primary ovarian insufficiency risk prediction model and 1,349 female SJLIFE survivors (101 with primary ovarian insufficiency) included in the external validation study.[62]
- The estimated primary ovarian insufficiency prevalence increased from 7.9% to 18.6% in the CCSS and 7.3% to 14.9% in the SJLIFE as survivors aged from 21 to 40 years. In patients who developed primary ovarian insufficiency, 531 of 922 (57.6%) in the CCSS and 50 of 101 (49.5%) in the SJLIFE had acute ovarian failure.
- The previous risk prediction model (that was limited to the period within 5 years of childhood cancer) was not useful for survivors whose menses resumed after treatment but had increased residual premature menopause risk by the time they were ready to have children. This study addressed this gap.
- This comprehensive model incorporated cumulative prescribed or delivered doses of abdominal and/or pelvic radiation therapy, HSCT information, and a range of chemotherapy agents, especially individual alkylators. The model worked well for both cohorts of survivors.
- This study evaluated genetic predictors, specifically a polygenic risk score consisting of genetic variants associated with treatment-related primary ovarian insufficiency in survivors.
- The online calculator is available on the CCSS website.
- A multicenter Dutch study evaluated treatment-related factors associated with abnormal hormonal and ultrasound markers of ovarian reserve in 5-year female childhood cancer survivors (diagnosed between 1963 and 2002) and in controls. There were 564 survivors and 390 controls who underwent clinical assessments of ovarian reserve, including antimüllerian hormone (AMH), FSH, inhibin B levels, and antral follicle counts (AFC).[63]
- Of the childhood cancer survivors, 7.0% to 17.7% had abnormal ovarian reserve markers, compared with 2.4% to 13.6% of controls.
- Significantly more childhood cancer survivors older than 35 years had abnormal ovarian reserve markers than did controls (AMH: 26% vs. 4%; AFC: 20% vs. 3%; inhibin B: 42% vs. 16%). AMH and FSH were also significantly different in survivors younger than 35 years.
- Treatments independently associated with at least one abnormal ovarian reserve marker included cyclophosphamide, procarbazine, a group of other alkylating agents, dactinomycin, doxorubicin, mitoxantrone, spinal radiation therapy, abdominal/pelvic radiation therapy, and TBI.
- Dose-effect relationships could only be established for procarbazine and abdominal/pelvic radiation therapy.
Fertility
Infertility remains one of the most common life-altering treatment effects experienced by long-term survivors of childhood cancer. Pediatric cancer cohort studies have demonstrated the impact of cytotoxic therapy on reproductive outcomes. CCSS investigations have elucidated factors contributing to subfertility among childhood cancer survivors.[64,65]
No abnormalities in fertility (reproductive characteristics and AMH levels as compared with controls) were identified in a series of 56 long-term female survivors of childhood differentiated thyroid cancer who received iodine I 131 (131I) for treatment. The median follow-up was 15.4 years (range, 8.3–24.7 years), and the median cumulative dose of 131I was 7.4 GBq/200.0 mCi. None of the survivors reported premature menopause.[66]
Evidence (excess risk of impaired fertility):
- Fertility was evaluated in 10,938 CCSS participants (5,640 males, 5,298 females) and 3,949 siblings.[64]
- At a median follow-up of 8 years from cohort entry, 38% of survivors reported having or siring a pregnancy, resulting in at least one live birth in 83% of those survivors.
- Among siblings monitored for a median of 10 years, 62% reported having or siring a pregnancy, resulting in at least one live birth in 90% of those siblings.
- Multivariable analysis confirmed that survivors had significantly decreased likelihood of siring or having a pregnancy (hazard ratio [HR], 0.63 in males and 0.87 in females) or of having a live birth (HR, 0.63 in males and 0.82 in females) than did siblings.
- Greater doses of alkylating drugs (HR, 0.82 per 5,000 mg/m2 increments) and cisplatin reduced the likelihood of siring pregnancy among male survivors, but only busulfan and higher doses (>411 mg/m2) of lomustine significantly reduced pregnancy among females.
- The risk of reduced likelihood of pregnancy in women was observed only at the highest cyclophosphamide equivalent dose (HR, 0.85 for upper quartile [≥11,295 mg/m2] vs. no exposure).
- HRs (95% CIs) for the likelihood of reporting first pregnancy by cyclophosphamide equivalent dose for male and female survivors are summarized in Table 16:
Table 16. Cyclophosphamide Equivalent Dose by Tertile and SexCyclophosphamide Equivalent Dose by Tertile | Male | Female |
---|
| HR (95% CI) | P Value | HR (95% CI) | P Value |
---|
CI = confidence interval; HR = hazard ratio. |
Lower (<4,897 mg/m2) | 1.14 (1.00–1.30) | .045 | 0.97 (0.86–1.08) | .55 |
Middle (4,897–9,638 mg/m2) | 0.79 (0.68–0.91) | .0010 | 0.98 (0.87–1.11) | .76 |
Upper (≥9,639 mg/m2) | 0.55 (0.47–0.64) | <.0001 | 0.90 (0.79–1.01) | .07 |
- Similar relationships were observed for live birth outcomes.
- Fertility may be impaired by factors other than the absence of sperm and ova. Conception requires delivery of sperm to the uterine cervix, patency of the fallopian tubes for fertilization to occur, and appropriate conditions in the uterus for implantation.
- Retrograde ejaculation occurs with a significant frequency in men who undergo bilateral retroperitoneal lymph node dissection.[8,9]
- Uterine structure may be affected by abdominal irradiation. A study demonstrated that uterine length was significantly shorter in ten women with ovarian failure who had been treated with whole-abdomen irradiation. Endometrial thickness did not increase in response to hormone replacement therapy in three women who underwent weekly ultrasonography examinations. Using Doppler ultrasonography, no blood flow was detectable through either uterine artery in five women, and through one uterine artery in three additional women.[67]
- In a study of menopausal status on reproductive outcomes in 2,930 survivors from the CCSS, investigators found that for those who ultimately developed nonsurgical premature menopause, rates of pregnancy and live birth were substantially reduced before nonsurgical premature menopause between the ages of 31 and 40 years. However, pregnancy and live birth rates did not differ for those aged 21 to 30 years on the basis of ultimate menopausal status.[53]
- Treatment variables significant for developing nonsurgical premature menopause by multivariable analyses included exposure to procarbazine doses higher than 4,000 mg/m2, any ovarian irradiation, and HSCT.
- A cyclophosphamide equivalent dose of 6,000 mg/m2 or higher that included procarbazine was significant in the univariate analysis, but it did not achieve significance in the multivariable analysis.
Sexual Function
The psychosexual health of adults who were treated for cancer during childhood, adolescence, and young adulthood has not been well studied.
An SJLIFE study estimated the prevalence of and risk factors for sexual dysfunction among 936 adult female survivors of childhood cancer. The study also evaluated associations between sexual dysfunction and psychological symptoms/quality of life.[68]
- Sexual dysfunction was prevalent in almost 20% of survivors. Survivors of germ cell tumors (OR, 8.8), renal tumors, (OR, 4.5) or leukemia (OR, 3.1) were at greater risk than controls.
- Older age at follow-up (45–54 vs. 18–24 years; OR, 5.7) and history of pelvic surgery (OR, 2.0), depression (OR, 2.0), and hypogonadism receiving hormone replacement (OR, 3.3) were associated with sexual dysfunction.
- Only 2.9% of survivors with sexual dysfunction reported receiving intervention.
Reproduction
Fertility preservation
Progress in reproductive endocrinology has resulted in the availability of several options for preserving or permitting fertility in patients about to receive potentially toxic chemotherapy or radiation therapy.[69,70,71,72]
- For males, cryopreservation of spermatozoa before treatment is an effective method to circumvent the sterilizing effect of therapy for all peripubertal and postpubertal males. Although pretreatment semen quality in patients with cancer has been shown to be less than that noted in healthy donors, the percentage decline in semen quality and the effect of cryodamage to spermatozoa from patients with cancer is similar to that of normal donors.[73,74]
- For males unable to bank sperm, newer technologies such as testicular sperm extraction may be an option. Further micromanipulative technological advances such as intracytoplasmic sperm injection and similar techniques may be able to render sperm extracted surgically, or even poor-quality cryopreserved spermatozoa from cancer patients, capable of successful fertilization.[75,76]
- Based on Norway national registries (Cancer Registry of Norway and Medical Birth Registry), young male cancer survivors were reported to be at a threefold increased risk of using assisted reproduction technology for offspring conception.[77]
- In a population-based nationwide study using the Swedish Medical Birth Registry, childhood cancer survivors and teenage and young adult cancer survivors were more likely to achieve paternity through intracytoplasmic sperm injection rather than in vitro fertilization.[78]
- For females, the most successful assisted-reproductive techniques depend on harvesting and banking the postpubertal patient's oocytes and cryopreserving unfertilized oocytes or embryos before gonadotoxic therapy.[79]
- Established options for females include ovarian transposition, shielding from radiation, and oocyte/embryo cryopreservation. Ovarian tissue cryopreservation is currently considered experimental in the United States, but it is performed as an established fertility preservation procedure in parts of Europe and Israel.[80,81] Options for prepubertal female patients are limited to investigational ovarian tissue cryopreservation for later autotransplant, which may be offered to girls with nonovarian, nonhematologic cancers.[82]
- Gonadotropin-releasing hormone agonists are used for ovarian suppression but are considered experimental, and their effectiveness is unproven.[83] Several randomized trials and meta-analyses have explored the benefits of gonadotropin-releasing hormone analogues during chemotherapy. However, the use of such analogues for ovarian protection during chemotherapy remains controversial. Two randomized controlled trials demonstrated that menstrual function, ovulation, and pregnancy were more likely to occur in patients with breast cancer after treatment with gonadotropin-releasing hormone agonists during chemotherapy, compared with those who did not receive this therapy. However, benefits for fertility outcomes are lacking. Studies have been limited by inadequate follow-up and the assessment of surrogate measures of fertility rather than pregnancy rates. While gonadotropin-releasing hormone analogues are not currently approved by the U.S. Food and Drug Administration for fertility preservation, these medications may be used off label.[84]
Risk of pregnancy complications
For survivors who maintain fertility, numerous investigations have evaluated the prevalence of and risk factors for pregnancy complications in adults treated for cancer during childhood. Pregnancy complications such as hypertension, fetal malposition, fetal loss/spontaneous abortion, preterm labor, and low birth weight have been observed in association with specific diagnostic and treatment groups.[85,86,87,88,89]
Evidence (excess risk of pregnancy complications):
- In a study of 4,029 pregnancies among 1,915 women monitored in the CCSS, there were 63% live births, 1% stillbirths, 15% miscarriages, 17% abortions, and 3% unknown or in gestation.[85]
- Risk of miscarriage was 3.6-fold higher in women treated with craniospinal irradiation and 1.7-fold higher in those treated with pelvic irradiation. Chemotherapy exposure alone did not increase risk of miscarriage.
- Survivors were less likely to have live births, more likely to have medical abortions, and more likely to have low-birth-weight babies than were siblings.
- Disruption of normal uterine function after radiation therapy or other treatment that results in reduced uterine volume and impaired uterine blood flow appears to be the underlying pathophysiology for many of these adverse obstetrical events.[90,91]
- In the National Wilms Tumor Study, records were obtained for 1,021 pregnancies of more than 20 weeks duration. In this group, there were 955 single live births.[92]
- Hypertension complicating pregnancy, early or threatened labor, malposition of the fetus, lower birth weight (<2,500 g), and premature delivery (<36 weeks) were more frequent among women who had received flank irradiation, in a dose-dependent manner.
- Another CCSS study evaluated pregnancy outcomes of partners of male survivors.[86]
- Among 4,106 sexually active males, 1,227 reported they sired 2,323 pregnancies, which resulted in 69% live births, 13% miscarriages, 13% abortions, and 5% unknown or in gestation at the time of analysis.
- Compared with partners of male siblings, there was a decreased incidence of live births (RR, 0.77), but no significant differences of pregnancy outcome by treatment.
- Results from a Danish study confirm the association of uterine irradiation with spontaneous abortion, but not other types of abortion. In the Danish Cancer Registry, more than 34,000 pregnancies were evaluated, including 1,479 pregnancies in 1,688 female survivors of childhood cancer. The pregnancy outcomes of survivors, 2,737 sisters, and 16,700 comparison women in the population were identified.[87]
- No significant differences were seen between survivors and comparison women in the proportions of live births, stillbirths, or all types of abortions combined.
- Survivors with a history of neuroendocrine or abdominal radiation therapy had an increased risk of spontaneous abortion.
- The pregnancy outcomes of survivors were similar to those of comparison women, with the exception of spontaneous abortion.
- In a retrospective cohort analysis from the CCSS of 1,148 men and 1,657 women who had survived cancer, there were 4,946 pregnancies.[88]
- Irradiation of the testes in men and pituitary gland in women and chemotherapy with alkylating drugs were not associated with an increased risk of stillbirth or neonatal death.
- Uterine and ovarian irradiation at doses higher than 10 Gy significantly increased the risk of stillbirth and neonatal death.
- For girls treated before menarche, irradiation of the uterus and ovaries at doses as low as 1 to 2.49 Gy significantly increased the risk of stillbirth or neonatal death.
- Most pregnancies reported by survivors who underwent HSCT and their partners result in live births.[89]
- In female HSCT survivors who were exposed to TBI, there appears to be an increased risk of preterm delivery of low-birth-weight infants.
- Female HSCT survivors are at higher risk of needing cesarean delivery than are the normal population (42% vs. 16%).
- A retrospective observational study included 178 women survivors of childhood leukemia from the long-term French follow-up program. The study examined women who had undergone prepubertal HSCT for acute leukemia. Among these survivors, 116 had received TBI and 62 had received a busulfan-based conditioning protocol. The median follow-up from HSCT was 18 years.[60]
- In this cohort, 22 women (12%) had at least one spontaneous pregnancy, for a total of 37. Of the 37 pregnancies, there were 17 live births, 14 miscarriages, 4 legal abortions, and 2 therapeutic abortions.
- Women who had not experienced premature ovarian insufficiency had better chances of spontaneous pregnancy than women who had immediate or secondary premature ovarian insufficiency.
- Preservation of fertility and successful pregnancies may occur after HSCT, although the conditioning regimens that include TBI, cyclophosphamide, and busulfan are highly gonadotoxic. One study evaluated pregnancy outcomes in a group of females treated with HSCT.[93]
- Among 708 women who were postpubertal at the time of transplant, 116 regained normal ovarian function and 32 became pregnant.
- Among 82 women who were prepubertal at the time of transplant, 23 had normal ovarian function and nine became pregnant.
- Of the 72 pregnancies in these 41 women, 16 occurred in those treated with TBI and 50% resulted in early termination.
- Among the 56 pregnancies in women treated with cyclophosphamide without either TBI or busulfan, 21% resulted in early termination.
- There were no pregnancies among the 73 women treated with busulfan and cyclophosphamide, and only one woman retained ovarian function.
- A German Pediatric Oncology Group study demonstrated that the rate of childbearing for female survivors of Hodgkin lymphoma was similar to that of the general population. However, the rate of childbearing was lower for survivors who received pelvic radiation therapy.[94]
- British CCSS investigators evaluated pregnancy and labor complications among female survivors of childhood cancer treated with abdominal radiation. The study linked British CCSS cohort data to a national hospital registry.[95]
- Survivors treated with abdominal radiation had a significantly higher risk (RR, 2.1) of pregnancy complications than did survivors who did not receive abdominal radiation.
- Risks were elevated for hypertension complicating pregnancy among Wilms tumor survivors (RR, 3.29) treated with abdominal radiation. Risks were also elevated for gestational diabetes mellitus (RR, 3.35) and anemia (RR, 2.10) among all survivors treated with abdominal radiation.
- A systematic review compared the data from published pregnancy and child health outcomes for pediatric and young adult leukemia and lymphoma survivors with the data from controls who did not have a history of cancer.[96]
- Compared with controls, survivors did not experience higher risks of spontaneous abortions, maternal diabetes and anemia, stillbirth, birth defects, or childhood cancer in offspring.
- Compared with controls, survivors had lower live birth rates and modestly higher risks of preterm birth and low birth weight.
- One study reviewed the effects of radiation therapy to the abdomen (partial or whole abdomen) on pregnancy and pregnancy outcomes in patients with a history of Wilms tumor. There were 1,021 pregnancies (with a duration of 20 weeks or longer), including 955 live births, from which there were 700 sets of maternal and offspring medical records.[92]
- Female survivors of Wilms tumor who received radiation therapy are at an increased risk of hypertension complicating pregnancy, early or threatened labor, and fetal malposition.
- The offspring of these patients were more likely to be premature and have low birth weights.
Offspring of childhood cancer survivors
For childhood cancer survivors who have offspring, there is concern about congenital anomalies, genetic disease, or risk of cancer in the offspring. Children of cancer survivors are not at significantly increased risk of congenital anomalies stemming from their parents' exposure to mutagenic cancer treatments.
Evidence (children of cancer survivors not at significantly increased risk of congenital anomalies):
- A retrospective cohort analysis of validated cases of congenital anomalies among 4,699 children of 1,128 male and 1,627 female participants of the CCSS observed the following:[97]
- No significant associations between gonadal radiation therapy or cumulative exposure to alkylating agents and congenital anomalies in offspring.
- A study compared 2,198 offspring of adult survivors treated for childhood cancer between 1945 and 1975 with 4,544 offspring of sibling controls.[98]
- There were no differences in the proportion of offspring with cytogenetic syndromes, single-gene defects, or simple malformations.
- There was similarly no effect of type of childhood cancer treatment on the occurrence of genetic disease in the offspring.
- A population-based study compared 2,630 live-born offspring of childhood cancer survivors with 5,504 live-born offspring of the survivors' siblings.[99]
- The study found no differences in proportion of abnormal karyotypes or incidence of Down syndrome or Turner syndrome between survivor and sibling offspring.
- In the same population-based cohort, survivors treated with abdominal radiation therapy and/or alkylating agents did not have an increased risk of offspring with genetic disease, compared with survivors not exposed to these agents.
- One study evaluated 5,847 offspring of survivors of childhood cancers treated in five Scandinavian countries.[100]
- In the absence of a hereditary cancer syndrome (such as hereditary retinoblastoma), there was no increased risk of cancer in offspring.
- Compared with the offspring of siblings, there was no excess risk of single-gene disorders, congenital malformations, or chromosomal syndromes among the offspring of former patients.[101]
- A European Group for Blood and Marrow Transplantation study evaluated pregnancy outcomes in 19,412 patients who underwent allogeneic transplant and 17,950 patients who underwent autologous transplant.[89]
- Investigators did not observe an increased risk of birth defects, developmental delay, or cancer among offspring of male and female HSCT recipients.
- A nationwide Finnish population-based registry study compared the risk of congenital anomalies in the offspring of 6,862 long-term survivors of childhood, adolescent, and young adult cancer treated between 1953 and 2004 with the risk of congenital anomalies in the offspring of 35,690 siblings.[102]
- Compared with siblings, childhood and adolescent survivors (prevalence ratio [PR], 1.17; 95% CI, 0.92–1.49) and young adult survivors (PR, 1.17; 95% CI, 0.83–1.23) were not at significant excess risk of congenital anomalies.
- There was an association between parent cancer and congenital anomalies in the offspring of survivors who were diagnosed in the earlier decades (1955–1964: PR, 2.77; 95% CI, 1.26–6.11; and 1965–1974: PR, 1.55; 95% CI, 0.94–2.56).
Table 17 summarizes reproductive late effects and the related health screenings.
Table 17. Reproductive Late Effectsa
Predisposing Therapy |
Reproductive Late Effects |
Health Screening |
AMH = antimüllerian hormone; FSH = follicle-stimulating hormone; LH = luteinizing hormone. |
a Adapted from theChildren's Oncology Group Long-Term Follow-Up Guidelines for Survivors of Childhood, Adolescent, and Young Adult Cancers. |
Alkylating agents; radiation exposing the gonads |
Testicular hormonal dysfunction: Testosterone deficiency/insufficiency; delayed/arrested puberty |
Tanner stage |
Morning testosterone |
LH |
Impaired spermatogenesis: Reduced fertility; oligospermia; azoospermia; infertility |
Semen analysis |
FSH |
Inhibin B |
Ovarian hormone deficiencies: Delayed/arrested puberty; premature ovarian insufficiency/premature menopause. Reduced ovarian follicular pool: Diminished ovarian reserve; infertility. |
Tanner stage |
Menstrual cycle history |
Estradiol |
FSH |
LH |
AMH |
Antral follicle count |
For reproductive late effects information, including risk factors, evaluation, and health counseling, see the Children's Oncology Group Long-Term Follow-Up Guidelines for Survivors of Childhood, Adolescent, and Young Adult Cancers.
References:
-
van Dorp W, Mulder RL, Kremer LC, et al.: Recommendations for Premature Ovarian Insufficiency Surveillance for Female Survivors of Childhood, Adolescent, and Young Adult Cancer: A Report From the International Late Effects of Childhood Cancer Guideline Harmonization Group in Collaboration With the PanCareSurFup Consortium. J Clin Oncol 34 (28): 3440-50, 2016.
-
Skinner R, Mulder RL, Kremer LC, et al.: Recommendations for gonadotoxicity surveillance in male childhood, adolescent, and young adult cancer survivors: a report from the International Late Effects of Childhood Cancer Guideline Harmonization Group in collaboration with the PanCareSurFup Consortium. Lancet Oncol 18 (2): e75-e90, 2017.
-
Green DM, Nolan VG, Goodman PJ, et al.: The cyclophosphamide equivalent dose as an approach for quantifying alkylating agent exposure: a report from the Childhood Cancer Survivor Study. Pediatr Blood Cancer 61 (1): 53-67, 2014.
-
Merchant TE, Wu S, Onar-Thomas A, et al.: Hypogonadism After Treatment for Medulloblastoma: Results From the SJMB03 Trial of Risk-Adapted Radiation Therapy. Int J Radiat Oncol Biol Phys 116 (3): 569-578, 2023.
-
Lehmann V, Chemaitilly W, Lu L, et al.: Gonadal Functioning and Perceptions of Infertility Risk Among Adult Survivors of Childhood Cancer: A Report From the St Jude Lifetime Cohort Study. J Clin Oncol 37 (11): 893-902, 2019.
-
Arap MA, Vicentini FC, Cocuzza M, et al.: Late hormonal levels, semen parameters, and presence of antisperm antibodies in patients treated for testicular torsion. J Androl 28 (4): 528-32, 2007 Jul-Aug.
-
Tryfonas G, Violaki A, Tsikopoulos G, et al.: Late postoperative results in males treated for testicular torsion during childhood. J Pediatr Surg 29 (4): 553-6, 1994.
-
Narayan P, Lange PH, Fraley EE: Ejaculation and fertility after extended retroperitoneal lymph node dissection for testicular cancer. J Urol 127 (4): 685-8, 1982.
-
Nijman JM, Jager S, Boer PW, et al.: The treatment of ejaculation disorders after retroperitoneal lymph node dissection. Cancer 50 (12): 2967-71, 1982.
-
Fankhauser CD, Afferi L, Stroup SP, et al.: Minimally invasive retroperitoneal lymph node dissection for men with testis cancer: a retrospective cohort study of safety and feasibility. World J Urol 40 (6): 1505-1512, 2022.
-
Schlegel PN, Walsh PC: Neuroanatomical approach to radical cystoprostatectomy with preservation of sexual function. J Urol 138 (6): 1402-6, 1987.
-
Frees S, Rubenwolf P, Ziesel C, et al.: Erectile function after treatment for rhabdomyosarcoma of prostate and bladder. J Pediatr Urol 12 (6): 404.e1-404.e6, 2016.
-
Hahn EW, Feingold SM, Simpson L, et al.: Recovery from aspermia induced by low-dose radiation in seminoma patients. Cancer 50 (2): 337-40, 1982.
-
Blatt J, Sherins RJ, Niebrugge D, et al.: Leydig cell function in boys following treatment for testicular relapse of acute lymphoblastic leukemia. J Clin Oncol 3 (9): 1227-31, 1985.
-
Izard MA: Leydig cell function and radiation: a review of the literature. Radiother Oncol 34 (1): 1-8, 1995.
-
Romerius P, Ståhl O, Moëll C, et al.: High risk of azoospermia in men treated for childhood cancer. Int J Androl 34 (1): 69-76, 2011.
-
Shafford EA, Kingston JE, Malpas JS, et al.: Testicular function following the treatment of Hodgkin's disease in childhood. Br J Cancer 68 (6): 1199-204, 1993.
-
Whitehead E, Shalet SM, Jones PH, et al.: Gonadal function after combination chemotherapy for Hodgkin's disease in childhood. Arch Dis Child 57 (4): 287-91, 1982.
-
Aubier F, Flamant F, Brauner R, et al.: Male gonadal function after chemotherapy for solid tumors in childhood. J Clin Oncol 7 (3): 304-9, 1989.
-
Jaffe N, Sullivan MP, Ried H, et al.: Male reproductive function in long-term survivors of childhood cancer. Med Pediatr Oncol 16 (4): 241-7, 1988.
-
Pryzant RM, Meistrich ML, Wilson G, et al.: Long-term reduction in sperm count after chemotherapy with and without radiation therapy for non-Hodgkin's lymphomas. J Clin Oncol 11 (2): 239-47, 1993.
-
Meistrich ML, Wilson G, Brown BW, et al.: Impact of cyclophosphamide on long-term reduction in sperm count in men treated with combination chemotherapy for Ewing and soft tissue sarcomas. Cancer 70 (11): 2703-12, 1992.
-
Kenney LB, Laufer MR, Grant FD, et al.: High risk of infertility and long term gonadal damage in males treated with high dose cyclophosphamide for sarcoma during childhood. Cancer 91 (3): 613-21, 2001.
-
Garolla A, Pizzato C, Ferlin A, et al.: Progress in the development of childhood cancer therapy. Reprod Toxicol 22 (2): 126-32, 2006.
-
Williams D, Crofton PM, Levitt G: Does ifosfamide affect gonadal function? Pediatr Blood Cancer 50 (2): 347-51, 2008.
-
Jahnukainen K, Heikkinen R, Henriksson M, et al.: Semen quality and fertility in adult long-term survivors of childhood acute lymphoblastic leukemia. Fertil Steril 96 (4): 837-42, 2011.
-
Green DM, Liu W, Kutteh WH, et al.: Cumulative alkylating agent exposure and semen parameters in adult survivors of childhood cancer: a report from the St Jude Lifetime Cohort Study. Lancet Oncol 15 (11): 1215-23, 2014.
-
Sanders JE, Buckner CD, Leonard JM, et al.: Late effects on gonadal function of cyclophosphamide, total-body irradiation, and marrow transplantation. Transplantation 36 (3): 252-5, 1983.
-
Viviani S, Santoro A, Ragni G, et al.: Gonadal toxicity after combination chemotherapy for Hodgkin's disease. Comparative results of MOPP vs ABVD. Eur J Cancer Clin Oncol 21 (5): 601-5, 1985.
-
Mackie EJ, Radford M, Shalet SM: Gonadal function following chemotherapy for childhood Hodgkin's disease. Med Pediatr Oncol 27 (2): 74-8, 1996.
-
Sherins RJ, Olweny CL, Ziegler JL: Gynecomastia and gonadal dysfunction in adolescent boys treated with combination chemotherapy for Hodgkin's disease. N Engl J Med 299 (1): 12-6, 1978.
-
Dhabhar BN, Malhotra H, Joseph R, et al.: Gonadal function in prepubertal boys following treatment for Hodgkin's disease. Am J Pediatr Hematol Oncol 15 (3): 306-10, 1993.
-
Heikens J, Behrendt H, Adriaanse R, et al.: Irreversible gonadal damage in male survivors of pediatric Hodgkin's disease. Cancer 78 (9): 2020-4, 1996.
-
da Cunha MF, Meistrich ML, Fuller LM, et al.: Recovery of spermatogenesis after treatment for Hodgkin's disease: limiting dose of MOPP chemotherapy. J Clin Oncol 2 (6): 571-7, 1984.
-
Brämswig JH, Heimes U, Heiermann E, et al.: The effects of different cumulative doses of chemotherapy on testicular function. Results in 75 patients treated for Hodgkin's disease during childhood or adolescence. Cancer 65 (6): 1298-302, 1990.
-
Green DM, Zhu L, Wang M, et al.: Effect of cranial irradiation on sperm concentration of adult survivors of childhood acute lymphoblastic leukemia: a report from the St. Jude Lifetime Cohort Study†. Hum Reprod 32 (6): 1192-1201, 2017.
-
Lopez R, Plat G, Bertrand Y, et al.: Testosterone deficiency in men surviving childhood acute leukemia after treatment with hematopoietic stem cell transplantation or testicular radiation: an L.E.A. study. Bone Marrow Transplant 56 (6): 1422-1425, 2021.
-
Cattoni A, Nicolosi ML, Capitoli G, et al.: Pubertal attainment and Leydig cell function following pediatric hematopoietic stem cell transplantation: a three-decade longitudinal assessment. Front Endocrinol (Lausanne) 14: 1292683, 2023.
-
Ishiguro H, Yasuda Y, Tomita Y, et al.: Gonadal shielding to irradiation is effective in protecting testicular growth and function in long-term survivors of bone marrow transplantation during childhood or adolescence. Bone Marrow Transplant 39 (8): 483-90, 2007.
-
Panasiuk A, Nussey S, Veys P, et al.: Gonadal function and fertility after stem cell transplantation in childhood: comparison of a reduced intensity conditioning regimen containing melphalan with a myeloablative regimen containing busulfan. Br J Haematol 170 (5): 719-26, 2015.
-
Green DM, Zhu L, Zhang N, et al.: Lack of specificity of plasma concentrations of inhibin B and follicle-stimulating hormone for identification of azoospermic survivors of childhood cancer: a report from the St Jude lifetime cohort study. J Clin Oncol 31 (10): 1324-8, 2013.
-
van Dorp W, van der Geest IM, Laven JS, et al.: Gonadal function recovery in very long-term male survivors of childhood cancer. Eur J Cancer 49 (6): 1280-6, 2013.
-
Chemaitilly W, Liu Q, van Iersel L, et al.: Leydig Cell Function in Male Survivors of Childhood Cancer: A Report From the St Jude Lifetime Cohort Study. J Clin Oncol 37 (32): 3018-3031, 2019.
-
Park JY, Kim DY, Suh DS, et al.: Outcomes of pediatric and adolescent girls with malignant ovarian germ cell tumors. Gynecol Oncol 137 (3): 418-22, 2015.
-
Wallace WH, Shalet SM, Crowne EC, et al.: Ovarian failure following abdominal irradiation in childhood: natural history and prognosis. Clin Oncol (R Coll Radiol) 1 (2): 75-9, 1989.
-
Scott JE: Pubertal development in children treated for nephroblastoma. J Pediatr Surg 16 (2): 122-5, 1981.
-
Hamre MR, Robison LL, Nesbit ME, et al.: Effects of radiation on ovarian function in long-term survivors of childhood acute lymphoblastic leukemia: a report from the Childrens Cancer Study Group. J Clin Oncol 5 (11): 1759-65, 1987.
-
Wallace WH, Shalet SM, Tetlow LJ, et al.: Ovarian function following the treatment of childhood acute lymphoblastic leukaemia. Med Pediatr Oncol 21 (5): 333-9, 1993.
-
Sklar CA, Mertens AC, Mitby P, et al.: Premature menopause in survivors of childhood cancer: a report from the childhood cancer survivor study. J Natl Cancer Inst 98 (13): 890-6, 2006.
-
Chemaitilly W, Mertens AC, Mitby P, et al.: Acute ovarian failure in the childhood cancer survivor study. J Clin Endocrinol Metab 91 (5): 1723-8, 2006.
-
Vatanen A, Wilhelmsson M, Borgström B, et al.: Ovarian function after allogeneic hematopoietic stem cell transplantation in childhood and adolescence. Eur J Endocrinol 170 (2): 211-8, 2014.
-
Sanders JE, Buckner CD, Amos D, et al.: Ovarian function following marrow transplantation for aplastic anemia or leukemia. J Clin Oncol 6 (5): 813-8, 1988.
-
Levine JM, Whitton JA, Ginsberg JP, et al.: Nonsurgical premature menopause and reproductive implications in survivors of childhood cancer: A report from the Childhood Cancer Survivor Study. Cancer 124 (5): 1044-1052, 2018.
-
van den Berg MH, van Dijk M, Byrne J, et al.: Treatment-related fertility impairment in long-term female childhood, adolescent and young adult cancer survivors: investigating dose-effect relationships in a European case-control study (PanCareLIFE). Hum Reprod 36 (6): 1561-1573, 2021.
-
van der Kaaij MA, Heutte N, Meijnders P, et al.: Premature ovarian failure and fertility in long-term survivors of Hodgkin's lymphoma: a European Organisation for Research and Treatment of Cancer Lymphoma Group and Groupe d'Etude des Lymphomes de l'Adulte Cohort Study. J Clin Oncol 30 (3): 291-9, 2012.
-
Thomas-Teinturier C, El Fayech C, Oberlin O, et al.: Age at menopause and its influencing factors in a cohort of survivors of childhood cancer: earlier but rarely premature. Hum Reprod 28 (2): 488-95, 2013.
-
Weil BR, Murphy AJ, Liu Q, et al.: Late Health Outcomes Among Survivors of Wilms Tumor Diagnosed Over Three Decades: A Report From the Childhood Cancer Survivor Study. J Clin Oncol 41 (14): 2638-2650, 2023.
-
Chemaitilly W, Li Z, Krasin MJ, et al.: Premature Ovarian Insufficiency in Childhood Cancer Survivors: A Report From the St. Jude Lifetime Cohort. J Clin Endocrinol Metab 102 (7): 2242-2250, 2017.
-
Bresters D, Emons JA, Nuri N, et al.: Ovarian insufficiency and pubertal development after hematopoietic stem cell transplantation in childhood. Pediatr Blood Cancer 61 (11): 2048-53, 2014.
-
Chabut M, Schneider P, Courbiere B, et al.: Ovarian Function and Spontaneous Pregnancy After Hematopoietic Stem Cell Transplantation for Leukemia Before Puberty: An L.E.A. Cohort Study. Transplant Cell Ther 29 (6): 378.e1-378.e9, 2023.
-
Clark RA, Mostoufi-Moab S, Yasui Y, et al.: Predicting acute ovarian failure in female survivors of childhood cancer: a cohort study in the Childhood Cancer Survivor Study (CCSS) and the St Jude Lifetime Cohort (SJLIFE). Lancet Oncol 21 (3): 436-445, 2020.
-
Im C, Lu Z, Mostoufi-Moab S, et al.: Development and validation of age-specific risk prediction models for primary ovarian insufficiency in long-term survivors of childhood cancer: a report from the Childhood Cancer Survivor Study and St Jude Lifetime Cohort. Lancet Oncol 24 (12): 1434-1442, 2023.
-
van den Berg MH, Overbeek A, Lambalk CB, et al.: Long-term effects of childhood cancer treatment on hormonal and ultrasound markers of ovarian reserve. Hum Reprod 33 (8): 1474-1488, 2018.
-
Chow EJ, Stratton KL, Leisenring WM, et al.: Pregnancy after chemotherapy in male and female survivors of childhood cancer treated between 1970 and 1999: a report from the Childhood Cancer Survivor Study cohort. Lancet Oncol 17 (5): 567-76, 2016.
-
Armuand G, Skoog-Svanberg A, Bladh M, et al.: Reproductive Patterns Among Childhood and Adolescent Cancer Survivors in Sweden: A Population-Based Matched-Cohort Study. J Clin Oncol 35 (14): 1577-1583, 2017.
-
Nies M, Cantineau AEP, Arts EGJM, et al.: Long-Term Effects of Radioiodine Treatment on Female Fertility in Survivors of Childhood Differentiated Thyroid Carcinoma. Thyroid 30 (8): 1169-1176, 2020.
-
Critchley HO, Wallace WH, Shalet SM, et al.: Abdominal irradiation in childhood; the potential for pregnancy. Br J Obstet Gynaecol 99 (5): 392-4, 1992.
-
Bjornard KL, Howell CR, Klosky JL, et al.: Psychosexual Functioning of Female Childhood Cancer Survivors: A Report From the St. Jude Lifetime Cohort Study. J Sex Med 17 (10): 1981-1994, 2020.
-
Loren AW, Mangu PB, Beck LN, et al.: Fertility preservation for patients with cancer: American Society of Clinical Oncology clinical practice guideline update. J Clin Oncol 31 (19): 2500-10, 2013.
-
Mulder RL, Font-Gonzalez A, Hudson MM, et al.: Fertility preservation for female patients with childhood, adolescent, and young adult cancer: recommendations from the PanCareLIFE Consortium and the International Late Effects of Childhood Cancer Guideline Harmonization Group. Lancet Oncol 22 (2): e45-e56, 2021.
-
Mulder RL, Font-Gonzalez A, Green DM, et al.: Fertility preservation for male patients with childhood, adolescent, and young adult cancer: recommendations from the PanCareLIFE Consortium and the International Late Effects of Childhood Cancer Guideline Harmonization Group. Lancet Oncol 22 (2): e57-e67, 2021.
-
Mulder RL, Font-Gonzalez A, van Dulmen-den Broeder E, et al.: Communication and ethical considerations for fertility preservation for patients with childhood, adolescent, and young adult cancer: recommendations from the PanCareLIFE Consortium and the International Late Effects of Childhood Cancer Guideline Harmonization Group. Lancet Oncol 22 (2): e68-e80, 2021.
-
Agarwa A: Semen banking in patients with cancer: 20-year experience. Int J Androl 23 (Suppl 2): 16-9, 2000.
-
Müller J, Sønksen J, Sommer P, et al.: Cryopreservation of semen from pubertal boys with cancer. Med Pediatr Oncol 34 (3): 191-4, 2000.
-
Dar S, Orvieto R, Levron J, et al.: IVF outcome in azoospermic cancer survivors. Eur J Obstet Gynecol Reprod Biol 220: 84-87, 2018.
-
Borgmann-Staudt A, Michael S, Sommerhaeuser G, et al.: The Use of Assisted Reproductive Technology by European Childhood Cancer Survivors. Curr Oncol 29 (8): 5748-5762, 2022.
-
Gunnes MW, Lie RT, Bjørge T, et al.: Reproduction and marriage among male survivors of cancer in childhood, adolescence and young adulthood: a national cohort study. Br J Cancer 114 (3): 348-56, 2016.
-
Kitlinski M, Giwercman A, Elenkov A: Paternity through use of assisted reproduction technology in male adult and childhood cancer survivors: a nationwide register study. Hum Reprod 38 (5): 973-981, 2023.
-
Domingo J, Ayllón Y, Domingo S, et al.: New approaches to female fertility preservation. Clin Transl Oncol 11 (3): 154-9, 2009.
-
Meirow D, Ra'anani H, Shapira M, et al.: Transplantations of frozen-thawed ovarian tissue demonstrate high reproductive performance and the need to revise restrictive criteria. Fertil Steril 106 (2): 467-74, 2016.
-
Pacheco F, Oktay K: Current Success and Efficiency of Autologous Ovarian Transplantation: A Meta-Analysis. Reprod Sci 24 (8): 1111-1120, 2017.
-
Oktay K, Karlikaya G: Ovarian function after transplantation of frozen, banked autologous ovarian tissue. N Engl J Med 342 (25): 1919, 2000.
-
Demeestere I, Brice P, Peccatori FA, et al.: No Evidence for the Benefit of Gonadotropin-Releasing Hormone Agonist in Preserving Ovarian Function and Fertility in Lymphoma Survivors Treated With Chemotherapy: Final Long-Term Report of a Prospective Randomized Trial. J Clin Oncol 34 (22): 2568-74, 2016.
-
Practice Committee of the American Society for Reproductive Medicine. Electronic address: asrm@asrm.org: Fertility preservation in patients undergoing gonadotoxic therapy or gonadectomy: a committee opinion. Fertil Steril 112 (6): 1022-1033, 2019.
-
Green DM, Kawashima T, Stovall M, et al.: Fertility of female survivors of childhood cancer: a report from the childhood cancer survivor study. J Clin Oncol 27 (16): 2677-85, 2009.
-
Green DM, Kawashima T, Stovall M, et al.: Fertility of male survivors of childhood cancer: a report from the Childhood Cancer Survivor Study. J Clin Oncol 28 (2): 332-9, 2010.
-
Winther JF, Boice JD, Svendsen AL, et al.: Spontaneous abortion in a Danish population-based cohort of childhood cancer survivors. J Clin Oncol 26 (26): 4340-6, 2008.
-
Signorello LB, Mulvihill JJ, Green DM, et al.: Stillbirth and neonatal death in relation to radiation exposure before conception: a retrospective cohort study. Lancet 376 (9741): 624-30, 2010.
-
Salooja N, Szydlo RM, Socie G, et al.: Pregnancy outcomes after peripheral blood or bone marrow transplantation: a retrospective survey. Lancet 358 (9278): 271-6, 2001.
-
Beneventi F, Locatelli E, Giorgiani G, et al.: Adolescent and adult uterine volume and uterine artery Doppler blood flow among subjects treated with bone marrow transplantation or chemotherapy in pediatric age: a case-control study. Fertil Steril 103 (2): 455-61, 2015.
-
van de Loo LEXM, van den Berg MH, Overbeek A, et al.: Uterine function, pregnancy complications, and pregnancy outcomes among female childhood cancer survivors. Fertil Steril 111 (2): 372-380, 2019.
-
Green DM, Lange JM, Peabody EM, et al.: Pregnancy outcome after treatment for Wilms tumor: a report from the national Wilms tumor long-term follow-up study. J Clin Oncol 28 (17): 2824-30, 2010.
-
Sanders JE, Hawley J, Levy W, et al.: Pregnancies following high-dose cyclophosphamide with or without high-dose busulfan or total-body irradiation and bone marrow transplantation. Blood 87 (7): 3045-52, 1996.
-
Brämswig JH, Riepenhausen M, Schellong G: Parenthood in adult female survivors treated for Hodgkin's lymphoma during childhood and adolescence: a prospective, longitudinal study. Lancet Oncol 16 (6): 667-75, 2015.
-
Reulen RC, Bright CJ, Winter DL, et al.: Pregnancy and Labor Complications in Female Survivors of Childhood Cancer: The British Childhood Cancer Survivor Study. J Natl Cancer Inst 109 (11): , 2017.
-
Shliakhtsitsava K, Romero SAD, Dewald SR, et al.: Pregnancy and child health outcomes in pediatric and young adult leukemia and lymphoma survivors: a systematic review. Leuk Lymphoma 59 (2): 381-397, 2018.
-
Signorello LB, Mulvihill JJ, Green DM, et al.: Congenital anomalies in the children of cancer survivors: a report from the childhood cancer survivor study. J Clin Oncol 30 (3): 239-45, 2012.
-
Winther JF, Boice JD, Mulvihill JJ, et al.: Chromosomal abnormalities among offspring of childhood-cancer survivors in Denmark: a population-based study. Am J Hum Genet 74 (6): 1282-5, 2004.
-
Winther JF, Olsen JH, Wu H, et al.: Genetic disease in the children of Danish survivors of childhood and adolescent cancer. J Clin Oncol 30 (1): 27-33, 2012.
-
Sankila R, Olsen JH, Anderson H, et al.: Risk of cancer among offspring of childhood-cancer survivors. Association of the Nordic Cancer Registries and the Nordic Society of Paediatric Haematology and Oncology. N Engl J Med 338 (19): 1339-44, 1998.
-
Byrne J, Rasmussen SA, Steinhorn SC, et al.: Genetic disease in offspring of long-term survivors of childhood and adolescent cancer. Am J Hum Genet 62 (1): 45-52, 1998.
-
Seppänen VI, Artama MS, Malila NK, et al.: Risk for congenital anomalies in offspring of childhood, adolescent and young adult cancer survivors. Int J Cancer 139 (8): 1721-30, 2016.
Late Effects of the Respiratory System
Respiratory function may be compromised in long-term survivors of childhood cancer who were treated with the following therapies:
- Specific chemotherapeutic agents.
- Thoracic radiation therapy.
- Pulmonary/chest wall surgery.
- Hematopoietic stem cell transplant (HSCT).
The effects of early lung injury from cancer treatment may be exacerbated by the decline in lung function associated with normal aging, other comorbid chronic health conditions, or smoking. The quality of current evidence regarding this outcome is limited by retrospective data collection, small sample size, cohort selection and participation bias, description of outcomes following antiquated treatment approaches, and variability in time since treatment and method of ascertainment. No large cohort studies have been performed that include clinical evaluations coupled with functional and quality-of-life assessments.
The true prevalence or incidence of pulmonary dysfunction in childhood and adolescent cancer survivors is not clear. For children treated with HSCT, significant clinical disease has been observed. Population-based studies have demonstrated that survivors experience excess morbidity and mortality related to respiratory conditions.[1,2]
- A study that linked population-based cancer registrations and inpatient admissions of 4,235 5-year childhood cancer survivors (aged 0–29 years) diagnosed between 1990 and 2011 compared admission rates of survivors with those of age- and sex-matched population controls.[1]
- The cumulative incidence for any type of respiratory conditions was 49% by age 40 years among survivors, which was 1.86 (95% confidence interval [CI], 1.73–2.01) times higher than for controls.
- Treatment with pulmonary toxic chemotherapy increased the risk of admission for all respiratory conditions (subdistribution hazard ratio (sHR), 1.26; 95% CI, 1.03–1.53) and pneumonia (sHR, 1.48; 95% CI, 1.01–2.17).
- Subsequent mortality was highest in patients admitted for pneumonia compared with other respiratory conditions (28% vs. 15%, respectively).
- Another population-based study investigated long-term respiratory mortality before age 40 years among 5-year cancer survivors participating in the British Childhood Cancer Survivor Study (BCCSS) and the Teenage and Young Adult Cancer Survivor Study (TYACSS).[2]
- Excess respiratory deaths were observed among survivors in the BCCSS (standardized mortality ratio [SMR], 6.8; 95% CI, 5.8–7.9) and TYACSS (SMR, 1.7; 95% CI, 1.6–1.8), with risks varying by type of respiratory death (e.g., pneumonia, pneumonitis, fibrosis, and chronic lower respiratory disease).
- Survivors of CNS tumors in the BCCSS and survivors of lung cancer, leukemia, and head and neck cancers in the TYACSS experienced the greatest number of excess respiratory deaths.
- An increase in excess number of respiratory deaths was noted with increasing age, but reassuringly, a decline in excess deaths was observed among more recently diagnosed survivors in both cohorts.
Evidence (selected cohort studies describing long-term pulmonary function outcomes):
- In a longitudinal study evaluating the magnitude and trajectory of pulmonary dysfunction among 121 childhood cancer survivors (median time from diagnosis to last evaluation, 17.1 years) treated with potentially pulmonary-toxic therapy (e.g., bleomycin, busulfan, pulmonary radiation therapy), survivors were significantly more likely to have restrictive and diffusion defects than were healthy controls.[3]
- Age younger than 16 years at diagnosis and exposure to more than 20 Gy of chest radiation were associated with increased odds of restrictive defects, whereas female sex and chest radiation dose were associated with diffusion abnormalities.
- Decline in pulmonary function over time was largely related to changes in diffusion capacity. The odds of decline in diffusion function over time showed a fourfold increase among females and 24-fold increase among survivors treated with more than 20 Gy of chest radiation. Compared with survivors with normal diffusion, those with diffusion defects were significantly more likely to be symptomatic and have poorer health-related quality-of-life scores, with decreases in the domains of physical functioning, role limitation because of physical health, and low energy/increased fatigue.
- Childhood Cancer Survivor Study (CCSS) investigators compared self-reported pulmonary outcomes and their impact on daily activities among 5-year cancer survivors (n = 14,316; median, 25 years from diagnosis) and a sibling cohort (n = 4,027).[4]
- Survivors were more likely to report chronic cough, the need for oxygen, lung fibrosis, and recurrent pneumonia than were siblings despite lower rates of smoking.
- By age 45 years, the cumulative incidence of any pulmonary condition was 29.6% for survivors. Survivors with chronic pulmonary conditions (e.g., chronic cough) were more likely to report activity limitations than were those without these conditions.
- Pulmonary complications contributed to an almost sixfold excess risk of death among survivors and demonstrated significant associations with exposure to platinum and lung radiation.
- St. Jude Life (SJLIFE) cohort study investigators evaluated risk factors for clinically ascertained pulmonary function deficits and the functional impact of pulmonary impairment in 606 adult survivors of childhood cancer (median age, 34 years at evaluation; median time from cancer diagnosis, 21.9 years).[5]
- Pulmonary function deficits were identified in the following:
- Forced expiratory volume in one second (FEV1) less than 80% predicted (50.7% of patients).
- Forced vital capacity (FVC) less than 80% predicted (47.2% of patients).
- Single-breath diffusing capacity of the lung for carbon monoxide (DLCO) corrected for hemoglobin less than 75% predicted (44.6% of patients).
- Obstructive lung defects (FEV1 /FVC, <0.7) were found in 0.8% of patients and restrictive defects (total lung capacity [TLC] <75%) in 31.2% of patients.
- Risk factors for abnormal pulmonary function included the following:
- Estimated percentage of lung tissue that received at least 10 Gy of radiation therapy (V10) and elapsed time from diagnosis for FEV1.
- Age at diagnosis and V10 for TLC.
- Increased body mass index, V10, and elapsed time from diagnosis for DLCO corrected for hemoglobin.
- Abnormal pulmonary function tests were associated with a decreased 6-minute walk distance.
Respiratory Complications After Radiation Therapy
Radiation therapy that exposes the lung parenchyma can result in pulmonary dysfunction related to reduced lung volume, impaired dynamic compliance, and deformity of both the lung and chest wall.
- The potential for chronic pulmonary sequelae is related to the radiation dose administered, the volume of lung irradiated, and the fractional radiation therapy doses.[6]
- Combined-modality therapy, including radiation therapy and pulmonary toxic chemotherapy or thoracic/chest wall surgery, increases the risk of pulmonary function impairment.[7,8]
- Chronic pulmonary complications reported after treatment for pediatric malignancies include restrictive or obstructive chronic pulmonary disease, pulmonary fibrosis, and spontaneous pneumothorax.[9]
These sequelae are uncommon after contemporary therapy, which most often results in subclinical injury that is detected only by imaging or formal pulmonary function testing.
The Pediatric Normal Tissue Effects in the Clinic (PENTEC) pulmonary task force reviewed dosimetric and clinical factors to develop predictive models for radiation therapy–associated pulmonary toxicity in children.[10]
- After partial lung radiation, acute pulmonary toxicity was uncommon, with grade 2 to 3 incidence of radiation pneumonitis of less than 1%.
- Late toxicities, including subclinical/asymptomatic impaired pulmonary function, were more common (<4%) and appeared to increase in incidence and severity over time.
- The risk of pulmonary complications was low among children treated with a mean lung dose of lower than 14 Gy and total lung V20Gy less than 30%.
- Within the spectrum of doses that could be analyzed, pulmonary toxicity was higher after whole-lung irradiation, but no dose-response relationship was identified.
- Bleomycin and other chemotherapeutic agents at current dose regimens did not contribute substantially to adverse pulmonary outcomes after partial lung irradiation. However, this risk increased with whole-lung irradiation.
Evidence (selected cohort studies describing pulmonary outcomes):
- For survivors of pediatric Hodgkin lymphoma, the prevalence of pulmonary symptoms using contemporary involved-field techniques is reported to be low. However, some patients exhibit substantial subclinical dysfunction.[11]
- A study of 54 children who were treated with induction chemotherapy (usually doxorubicin, bleomycin, vincristine, etoposide, prednisone, and cyclophosphamide–based chemotherapy) and 21 Gy of radiation therapy from 2008 through 2016 confirmed these findings.[12]
- At a median follow-up of 39.5 months, 3 of 54 patients (5.6%) or 3 of 108 lungs (2.8%) developed radiation pneumonitis (RP); two lungs had grade 1 RP and one lung had grade 2 RP.
- RP was seen only in patients with a median lung dose of higher than 12.4 Gy or with an individual mean lung dose higher than 13.8 Gy.
- In a study of 109 patients (59% with Hodgkin lymphoma) who were irradiated at a median age of 13.8 years (range, 0.04–20.9 years; median follow-up, 3.4 years), patients were treated with a median prescribed radiation therapy dose of 21 Gy (range, 0.4–64.8 Gy); 58.7% of patients received bleomycin, and 83.5% of patients received cyclophosphamide.[13]
- The 5-year cumulative incidence after irradiation was: pneumonitis, 6%; chronic cough, 10%; pneumonia, 35%; dyspnea, 11%; supplemental oxygen requirement, 2%; radiographic interstitial lung disease, 40%; and chest wall deformity, 12%.
- One patient died of progressive respiratory failure.
- Postirradiation pulmonary function tests available from 44 patients showed evidence of obstructive lung disease (25%), restrictive disease (11%), hyperinflation (32%), and abnormal diffusion capacity (12%).
- Thoracic surgery, bleomycin, age, maximum lung irradiation dose V30 (volume of lung exposed to a radiation dose >30 Gy), and volume of lung exposed to a radiation dose of greater than 25 Gy were significant risk factors for the development of adverse pulmonary outcomes on univariate analysis.
- Mean lung irradiation dose, maximum lung dose, and Vdose (percentage of volume of lung receiving the threshold dose or greater) were highly correlated. On multivariate analysis, mean lung irradiation dose was the sole significant predictor of adverse pulmonary outcome (P = .01).
- Changes in lung function have been reported in children treated with whole-lung radiation therapy for metastatic Wilms tumor.[14,15]
- A dose of 12 Gy to 14 Gy reduced TLC and vital capacity to about 70% of predicted values, and even lower if the patient had undergone thoracotomy.
- Administration of bleomycin alone can produce pulmonary toxicity and, when combined with radiation therapy, can heighten radiation reactions. Chemotherapeutic agents such as doxorubicin, dactinomycin, and busulfan are radiomimetic agents and can reactivate underlying radiation damage.[14,15]
Respiratory Complications After Chemotherapy
Chemotherapy agents with potential pulmonary toxic effects commonly used in the treatment of pediatric malignancies include bleomycin, busulfan, and the nitrosoureas (carmustine and lomustine). These agents induce lung damage on their own or potentiate the damaging effects of radiation to the lung.
Combined-modality therapy, including pulmonary toxic chemotherapy and thoracic radiation therapy or thoracic/chest wall surgery, increases the risk of pulmonary function impairment.[7]
Evidence (outcomes among cohorts treated with pulmonary toxic chemotherapy):
- The development of bleomycin-associated pulmonary fibrosis with permanent restrictive disease is dose dependent, usually occurring at doses greater than 200 U/m2 to 400 U/m2, higher than those used in treatment protocols for pediatric malignancies.[16,17]
- Pediatric regimens for Hodgkin lymphoma using radiation therapy and doxorubicin, bleomycin, vinblastine, and dacarbazine (ABVD) have shown a significant incidence of asymptomatic pulmonary dysfunction after treatment, which appears to improve with time.[18] However, grades 3 and 4 pulmonary toxicity was reported in 9% of children receiving 12 cycles of ABVD followed by 21 Gy of extended-field radiation.[17]
- ABVD-related pulmonary toxic effects may result from fibrosis induced by bleomycin or radiation recall pneumonitis related to administration of doxorubicin.
- One series included 207 children who were treated with bleomycin for Hodgkin lymphoma or germ cell tumors and had pulmonary function tests at least 1 year after the completion of therapy. At least one pulmonary function abnormality was present in 52.5% of patients. Obstructive lung disease was noted in 22.7% of patients, restrictive lung disease in 7.5%, hyperinflation in 28.8%, and nonuniform ventilation in 14%. Very few patients had pulmonary symptoms.[19]
- Pulmonary veno-occlusive disease has been observed rarely and has been attributed to bleomycin or alkylating agents.[20,21]
Respiratory Complications Associated With HSCT
- Patients undergoing HSCT are at increased risk of pulmonary toxic effects related to the following:[22]
- Preexisting pulmonary dysfunction (e.g., asthma, pretransplant therapy).
- Conditioning regimens, including cyclophosphamide, busulfan, or carmustine.
- Total-body irradiation (TBI).
- Graft-versus-host disease (GVHD).
- Although most survivors of transplant are not clinically compromised, restrictive lung disease may occur and has been reported to increase in prevalence with increasing time from HSCT, based on limited data from longitudinally followed cohorts.[23,24]
- Obstructive disease is less common, as is late-onset pulmonary syndrome, which includes the spectrum of restrictive and obstructive disease.
- Restrictive or interstitial lung disease has been reported in survivors of high-risk neuroblastoma who underwent an HSCT (with or without TBI). In a series of 48 survivors, radiation therapy did not have a direct correlation with pulmonary function tests, while cyclophosphamide and ifosfamide did affect pulmonary function tests. A higher median dose of cyclophosphamide was associated with restrictive lung disease, whereas higher doses of ifosfamide correlated with both restrictive lung disease and interstitial lung disease. In this cohort, 25% of the patients were symptomatic, but not all of these symptoms correlated with abnormal pulmonary function tests.[25]
- Bronchiolitis obliterans with or without organizing pneumonia, diffuse alveolar damage, and interstitial pneumonia may occur as a component of this syndrome, generally between 6 and 12 months posttransplant. Cough, dyspnea, or wheezing may occur with either normal chest x-ray or diffuse/patchy infiltrates; however, most patients are symptom free.[26,27]
Other Factors Associated With Respiratory Late Effects
- Additional factors contributing to chronic pulmonary toxic effects include superimposed infection, underlying pneumonopathy (e.g., asthma), chest wall abnormalities, respiratory toxic effects, chronic GVHD, and the effects of chronic pulmonary involvement by tumor or reaction to tumor.[8]
- Lung lobectomy during childhood appears to have no significant impact on long-term pulmonary function,[28] but the long-term effect of lung surgery for children with cancer is not well defined.
- Pulmonary complications may also be exacerbated by smoking cigarettes or other substances. While smoking rates in survivors of childhood cancer tend to be lower than the general population, it is still important to prevent initiation of smoking and promote cessation in this distinct population.[29]
Evidence (pulmonary dysfunction in former or current smokers):
- Pulmonary function evaluations of 433 adult survivors of childhood cancer who were treated with pulmonary toxic modalities demonstrated significantly higher risk of pulmonary dysfunction in smokers than in nonsmokers.[30]
- FEV1 /FVC median values among current and former smokers were lower than those who had never smoked.
- Median FEV1 /FVC values were lower among those who smoked less than 6 pack-years and those who smoked 6 pack-years or more compared with those who had never smoked. This suggests that survivors who are former or current smokers have an increased risk of future obstructive and restrictive lung disease.
Table 18 summarizes respiratory late effects and the related health screenings.
Table 18. Respiratory Late Effectsa
Predisposing Therapy |
Respiratory Effects |
Health Screening/Interventions |
DLCO = diffusing capacity of the lung for carbon monoxide; GVHD = graft-versus-host disease; HSCT = hematopoietic stem cell transplant. |
a Adapted from theChildren's Oncology Group Long-Term Follow-Up Guidelines for Survivors of Childhood, Adolescent, and Young Adult Cancers. |
Busulfan; carmustine (BCNU)/lomustine (CCNU); bleomycin; radiation exposing lungs; surgery impacting pulmonary function (lobectomy, metastasectomy, wedge resection) |
Subclinical pulmonary dysfunction; interstitial pneumonitis; pulmonary fibrosis; restrictive lung disease; obstructive lung disease |
History: cough, shortness of breath, dyspnea on exertion, wheezing |
Pulmonary examination |
Pulmonary function tests (including DLCO and spirometry) |
Chest x-ray |
Counsel regarding tobacco avoidance/smoking cessation |
In patients with abnormal pulmonary function tests and/or chest x-ray, consider repeat evaluation before general anesthesia |
Pulmonary consultation for patients with symptomatic pulmonary dysfunction |
Influenza and pneumococcal vaccinations |
HSCT with any history of chronic GVHD |
Pulmonary toxicity (bronchiolitis obliterans, chronic bronchitis, bronchiectasis) |
History: cough, shortness of breath, dyspnea on exertion, wheezing |
Pulmonary examination |
Pulmonary function tests (including DLCO and spirometry) |
Chest x-ray |
Counsel regarding tobacco avoidance/smoking cessation |
In patients with abnormal pulmonary function tests and/or chest x-ray, consider repeat evaluation before general anesthesia |
Pulmonary consultation for patients with symptomatic pulmonary dysfunction |
Influenza and pneumococcal vaccinations |
For respiratory late effects information, including risk factors, evaluation, and health counseling, see the Children's Oncology Group Long-Term Follow-Up Guidelines for Survivors of Childhood, Adolescent, and Young Adult Cancers.
References:
-
Smith L, Glaser AW, Peckham D, et al.: Respiratory morbidity in young people surviving cancer: Population-based study of hospital admissions, treatment-related risk factors and subsequent mortality. Int J Cancer 145 (1): 20-28, 2019.
-
Fidler MM, Reulen RC, Bright CJ, et al.: Respiratory mortality of childhood, adolescent and young adult cancer survivors. Thorax 73 (10): 959-968, 2018.
-
Armenian SH, Landier W, Francisco L, et al.: Long-term pulmonary function in survivors of childhood cancer. J Clin Oncol 33 (14): 1592-600, 2015.
-
Dietz AC, Chen Y, Yasui Y, et al.: Risk and impact of pulmonary complications in survivors of childhood cancer: A report from the Childhood Cancer Survivor Study. Cancer 122 (23): 3687-3696, 2016.
-
Green DM, Zhu L, Wang M, et al.: Pulmonary Function after Treatment for Childhood Cancer. A Report from the St. Jude Lifetime Cohort Study (SJLIFE). Ann Am Thorac Soc 13 (9): 1575-85, 2016.
-
Huang TT, Hudson MM, Stokes DC, et al.: Pulmonary outcomes in survivors of childhood cancer: a systematic review. Chest 140 (4): 881-901, 2011.
-
Mulder RL, Thönissen NM, van der Pal HJ, et al.: Pulmonary function impairment measured by pulmonary function tests in long-term survivors of childhood cancer. Thorax 66 (12): 1065-71, 2011.
-
Kasteler R, Weiss A, Schindler M, et al.: Long-term pulmonary disease among Swiss childhood cancer survivors. Pediatr Blood Cancer 65 (1): , 2018.
-
Josephson MB, Goldfarb SB: Pulmonary complications of childhood cancers. Expert Rev Respir Med 8 (5): 561-71, 2014.
-
Briere TM, Agrusa JE, Martel MK, et al.: Acute and Late Pulmonary Effects After Radiation Therapy in Childhood Cancer Survivors: A PENTEC Comprehensive Review. Int J Radiat Oncol Biol Phys 119 (2): 533-548, 2024.
-
Venkatramani R, Kamath S, Wong K, et al.: Pulmonary outcomes in patients with Hodgkin lymphoma treated with involved field radiation. Pediatr Blood Cancer 61 (7): 1277-81, 2014.
-
Lewis GD, Agrusa JE, Teh BS, et al.: Radiation pneumonitis in pediatric Hodgkin lymphoma patients receiving radiation therapy to the chest. Pract Radiat Oncol 8 (5): e364-e368, 2018 Sep - Oct.
-
Venkatramani R, Kamath S, Wong K, et al.: Correlation of clinical and dosimetric factors with adverse pulmonary outcomes in children after lung irradiation. Int J Radiat Oncol Biol Phys 86 (5): 942-8, 2013.
-
McDonald S, Rubin P, Maasilta P: Response of normal lung to irradiation. Tolerance doses/tolerance volumes in pulmonary radiation syndromes. Front Radiat Ther Oncol 23: 255-76; discussion 299-301, 1989.
-
McDonald S, Rubin P, Phillips TL, et al.: Injury to the lung from cancer therapy: clinical syndromes, measurable endpoints, and potential scoring systems. Int J Radiat Oncol Biol Phys 31 (5): 1187-203, 1995.
-
Bossi G, Cerveri I, Volpini E, et al.: Long-term pulmonary sequelae after treatment of childhood Hodgkin's disease. Ann Oncol 8 (Suppl 1): 19-24, 1997.
-
Fryer CJ, Hutchinson RJ, Krailo M, et al.: Efficacy and toxicity of 12 courses of ABVD chemotherapy followed by low-dose regional radiation in advanced Hodgkin's disease in children: a report from the Children's Cancer Study Group. J Clin Oncol 8 (12): 1971-80, 1990.
-
Hudson MM, Greenwald C, Thompson E, et al.: Efficacy and toxicity of multiagent chemotherapy and low-dose involved-field radiotherapy in children and adolescents with Hodgkin's disease. J Clin Oncol 11 (1): 100-8, 1993.
-
De A, Guryev I, LaRiviere A, et al.: Pulmonary function abnormalities in childhood cancer survivors treated with bleomycin. Pediatr Blood Cancer 61 (9): 1679-84, 2014.
-
Polliack A: Late therapy-induced cardiac and pulmonary complications in cured patients with Hodgkin's disease treated with conventional combination chemo-radiotherapy. Leuk Lymphoma 15 (Suppl 1): 7-10, 1995.
-
Ranchoux B, Günther S, Quarck R, et al.: Chemotherapy-induced pulmonary hypertension: role of alkylating agents. Am J Pathol 185 (2): 356-71, 2015.
-
Marras TK, Chan CK, Lipton JH, et al.: Long-term pulmonary function abnormalities and survival after allogeneic marrow transplantation. Bone Marrow Transplant 33 (5): 509-17, 2004.
-
Inaba H, Yang J, Pan J, et al.: Pulmonary dysfunction in survivors of childhood hematologic malignancies after allogeneic hematopoietic stem cell transplantation. Cancer 116 (8): 2020-30, 2010.
-
Frisk P, Arvidson J, Hedenström H: A longitudinal study of pulmonary function after stem cell transplantation, from childhood to young adulthood. Pediatr Blood Cancer 58 (5): 775-9, 2012.
-
Hobbie WL, Li Y, Carlson C, et al.: Late effects in survivors of high-risk neuroblastoma following stem cell transplant with and without total body irradiation. Pediatr Blood Cancer 69 (3): e29537, 2022.
-
Uderzo C, Pillon M, Corti P, et al.: Impact of cumulative anthracycline dose, preparative regimen and chronic graft-versus-host disease on pulmonary and cardiac function in children 5 years after allogeneic hematopoietic stem cell transplantation: a prospective evaluation on behalf of the EBMT Pediatric Diseases and Late Effects Working Parties. Bone Marrow Transplant 39 (11): 667-75, 2007.
-
Yoshihara S, Yanik G, Cooke KR, et al.: Bronchiolitis obliterans syndrome (BOS), bronchiolitis obliterans organizing pneumonia (BOOP), and other late-onset noninfectious pulmonary complications following allogeneic hematopoietic stem cell transplantation. Biol Blood Marrow Transplant 13 (7): 749-59, 2007.
-
Kreisel D, Krupnick AS, Huddleston CB: Outcomes and late complications after pulmonary resections in the pediatric population. Semin Thorac Cardiovasc Surg 16 (3): 215-9, 2004.
-
Gibson TM, Liu W, Armstrong GT, et al.: Longitudinal smoking patterns in survivors of childhood cancer: An update from the Childhood Cancer Survivor Study. Cancer 121 (22): 4035-43, 2015.
-
Oancea SC, Gurney JG, Ness KK, et al.: Cigarette smoking and pulmonary function in adult survivors of childhood cancer exposed to pulmonary-toxic therapy: results from the St. Jude lifetime cohort study. Cancer Epidemiol Biomarkers Prev 23 (9): 1938-43, 2014.
Late Effects of the Special Senses
Hearing
Hearing loss as a late effect of therapy can occur after exposure to platinum compounds (cisplatin and carboplatin), cranial radiation therapy, or both. These therapeutic exposures are most common in the treatment of central nervous system (CNS) and non-CNS solid tumors. Children are more susceptible to otologic toxic effects from platinum agents than adults.[1]
Evidence (hearing loss):
- A longitudinal study evaluated the incidence of and risk factors for hearing loss in 171 patients (340 ears) with CNS and head and neck tumors treated with multiple ototoxic exposures. The study reported associations with severe hearing loss, ototoxic chemotherapy, and cochlea dose.[2]
- Mean cochlea dose (odds ratio [OR], 1.04 per Gy; P < .001), time since radiation therapy (OR, 1.21 per year; P < .001), cisplatin dose (OR, 1.48 per 100 mg/m2; P < .001), and carboplatin dose (OR, 1.41 per 1,000 mg/m2; P = .002) were associated with increasing grade of hearing loss, according to the International Society of Pediatric Oncology (SIOP)-Boston ototoxicity scale.
- The cumulative incidence of high-frequency hearing loss (>4 kHz) was 50% or greater at 5 years after radiation if mean cochlea dose was higher than 30 Gy.
- The incidence of hearing loss across all frequencies continued to increase beyond 5 years after radiation therapy.
- There was no synergistic effect of radiation therapy and cisplatin (interaction term, P = .53) or radiation therapy and carboplatin (interaction term, P = .85).
- A report from the Swiss Childhood Cancer Survivor Study (CCSS) (N = 2,061) estimated the prevalence of hearing loss in survivors at 10%, compared with 3% in siblings. Hearing loss was most common in survivors of CNS tumors (25%), neuroblastomas (23%), hepatic tumors (21%), germ cell tumors (20%), bone tumors (16%), and soft tissue sarcomas (16%).[3]
- A follow-up study from this cohort was limited to 270 childhood cancer survivors treated with platinum-based chemotherapy. Varying doses of cisplatin were used. Of those who received cisplatin, the median cumulative dose was 400 mg/m2 (interquartile range, 314–480 mg/m2). Hearing loss, assessed using audiological evaluations, was characterized as mild (20%), moderate (29%), and severe (28%). More severe hearing loss was associated with younger age at diagnosis (OR, 5.4 for <5 years), treatment in earlier years (OR, 4.8 for 1990–1995), higher cisplatin doses (OR, 13.5 for >450 mg/m2), concomitant cranial radiation therapy (OR, 4.4), and hematopoietic stem cell transplant (HSCT) (OR, 2.7).[4]
- A population-based study used health registry data to evaluate the long-term incidence and predictors of hearing loss requiring hearing amplification devices (HAD) among Canadian childhood cancer survivors.[5]
- Among 11,842 cases and 59,210 controls identified, cases were at higher risk of needing an HAD (hazard ratio [HR], 12.8; 95% confidence interval [CI], 9.8–16.7).
- The cumulative incidence of HAD among survivors was 2.1% (95% CI, 1.7%–2.5%) at 20 years and increased to 6.4% (95% CI, 2.8%–12.1%) at 30 years postdiagnosis.
- The 30-year incidence was highest in survivors of neuroblastoma (10.7%; 95% CI, 3.8%–21.7%) and hepatoblastoma (16.2%; 95% CI, 8.6%–26.0%).
- In multivariable analyses, predictors of requiring an HAD included the following:
- Age 0 to 4 years at diagnosis versus age 5 to 9 years (HR, 2.2; 95% CI, 1.4–3.3).
- Treatment with 200 mg/m2 or more of cisplatin versus no cisplatin (HR, 3.1; 1.8–5.5).
- Treatment with cranial or facial radiation therapy of higher than 32 Gy versus radiation therapy of 32 Gy or lower (HR, 2.4; 1.6–3.7).
- Data from the North American CCSS indicated that the relative rate of first occurrence of auditory complications (i.e., problems hearing sounds, tinnitus, hearing loss, deafness) is greatest in the time period from diagnosis to 5 years postdiagnosis. However, during the period of 5 or more years postdiagnosis, the risk of developing such conditions for survivors remained significantly higher than for siblings.[6]
Risk factors associated with hearing loss include the following:
- Younger age at treatment.[5,7]
- Higher cumulative dose of platinum-based chemotherapy (≥200 mg/m2).[5,8] Based on the results of a multi-institutional North American cohort study (n = 1,481), significant risk factors for developing cisplatin-induced hearing loss included both the cumulative dose of cisplatin and how the cisplatin was administered. Higher fractionated doses were associated with risk of cisplatin-induced hearing loss (for each 10 mg/m2 increase/day: adjusted OR, 1.15; for each 50 mg/m2 increase per cycle: adjusted OR, 2.16).[7]
- Exposure to cisplatin combined with carboplatin.[8]
- CNS tumors.[7]
- Cranial radiation therapy.[5,7]
- Neurosurgery.
Hearing loss and platinum-based therapy
- Platinum-related sensorineural hearing loss develops as an acute toxicity that is generally irreversible and bilateral.[9]
- The prevalence of hearing loss has varied widely per series and is based on platinum treatment (e.g., platinum type, dose, infusion duration); host factors (e.g., age, genetic susceptibility, renal function); receipt of additional ototoxic therapy (cranial radiation therapy, aminoglycosides, loop diuretics), and the grading criteria used to report prevalence and severity of hearing loss.[8,9,10]
- Hearing loss manifests initially in the high frequencies and progresses to the speech frequencies with increasing cumulative exposure.[9]
- Cisplatin-induced hearing loss involving the speech frequencies (500–2,000 Hz) usually occurs with cumulative doses that exceed 400 mg/m2 in pediatric patients.[11,12]
- Prolonging the duration of infusion or splitting the dose has been reported to reduce the risk of significant hearing loss.[13]
- In two randomized trials (Children's Oncology Group ACCL0431 [NCT00716976] and International Childhood Liver Tumour Strategy Group SIOPEL-6 [NCT00652132]), sodium thiosulfate demonstrated efficacy in preventing cisplatin-induced hearing loss.[14,15]
The SIOPEL-6 trial compared cisplatin alone with cisplatin plus delayed administration of sodium thiosulfate (sodium thiosulfate administered 6 hours after cisplatin) resulted in a 48% lower incidence of cisplatin-induced hearing loss among children with standard-risk hepatoblastoma. Sodium thiosulfate treatment did not jeopardize the overall survival or event-free survival.[15] These trials led to regulatory approval of sodium thiosulfate for prevention of cisplatin-induced hearing loss. However, each trial used a different hearing end point. In 2010, the SIOP-Boston ototoxicity scale was developed to overcome key limitations of the available scales, and it is central to harmonizing hearing end points. Using the SIOP scale, hearing outcomes from the ACCL0431 trial were re-evaluated. This analysis showed that children treated with sodium thiosulfate were approximately 90% less likely to develop higher than grade 2 cisplatin-induced hearing loss during the trial period or at the end of cisplatin therapy, compared with the observation group.[16]
- Otologic toxic effects after platinum chemotherapy have been reported to worsen years after completion of therapy.[17]
- Radiation therapy to the posterior fossa inclusive of the eighth cranial nerve (suggestive of damage to the cochlea at the end of therapy) increases the risk of late-onset hearing loss in survivors treated with cisplatin.[18]
- Carboplatin used in conventional (nonmyeloablative) dosing is typically not ototoxic.[19] However, delayed-onset hearing loss has been reported in the following populations:
- In a cross-sectional, multicenter analysis that included 451 Dutch childhood cancer survivors who received platinum agents but not cranial radiation therapy, the incidence of ototoxicity (defined as Münster grade >2b [>20 dB at ≥4–8kHz]) associated with the use of carboplatin alone (n = 112) was 17%.[8]
- A single study of otologic toxic effects after non-HSCT dosing of carboplatin for retinoblastoma reported that 8 of 175 children developed hearing loss. For seven of the eight children, the onset of the otologic toxic effects was delayed a median of 3.7 years.[20]
- Another study that evaluated audiological outcomes among 60 retinoblastoma survivors treated with nonmyeloablative systemic carboplatin and vincristine estimated a cumulative incidence of hearing loss of 20.3% at 10 years.[21]
- Among the ten patients (17%) who developed sustained grade 3 or grade 4 hearing loss, nine were younger than 6 months at the start of chemotherapy.
- Younger age at the start of treatment was the only significant predictor of hearing loss. The cumulative incidence of hearing loss was 39% for patients younger than 6 months versus only 8.3% for patients aged 6 months and older.
- The use of a carboplatin conditioning regimen for HSCT, particularly in combination with previous carboplatin or cisplatin therapy, may cause significant otologic toxic effects.[11,12]
- One series evaluated 115 patients with recurrent or refractory germ cell tumors (median age, 32 years) who had received first-line cisplatin-based chemotherapy. The study reported hearing outcomes for adolescents and young adults treated with three cycles of high-dose carboplatin therapy. Of the 106 patients with normal hearing or mild hearing loss before high-dose carboplatin therapy, 70 (66%) developed moderate-to-profound hearing loss in the speech frequencies after high-dose carboplatin therapy. Twenty-five patients (22%) were recommended for hearing aids after high-dose carboplatin therapy. Patients with moderate-to-profound hearing loss isolated to higher frequencies before high-dose carboplatin therapy were more likely to develop moderate-to-profound hearing loss in the speech frequencies after therapy and be recommended for hearing aids.[22]
Hearing loss and cranial radiation therapy
- Cranial radiation therapy, when used as a single modality, may result in otologic toxic effects that may be gradual in onset, manifesting months to years after exposure. The threshold dose for auditory toxicity after radiation therapy alone is in the range of 35 Gy to 45 Gy for children.[23]
- High-frequency sensorineural hearing loss is uncommon at cumulative radiation doses below 35 Gy, and is rarely severe below doses of 45 Gy.[24] The exception is for patients with supratentorial tumors and ventriculoperitoneal shunts, in whom doses below 30 Gy may be associated with intermediate frequency (1,000–2,000 Hz) hearing loss.[23,25]
- To reduce the risk of hearing loss, the average cochlear dose should not exceed 30 Gy to 35 Gy, delivered over 6 weeks.
- Sensorineural hearing loss after cranial radiation therapy can progress over time.
- A study included 235 pediatric patients with brain tumors who were treated with conformal or intensity-modulated radiation therapy (without cisplatin or preexisting hearing loss) and monitored for a median of 9 years. Sensorineural hearing loss was prevalent in 14% of patients, with a median time to onset of 3.6 years from radiation therapy.[26]
- Follow-up evaluations among 29 patients identified continued decline in hearing sensitivity.
- Risk factors for cranial radiation–associated sensorineural hearing loss included younger age at initiation of radiation, higher cochlear radiation dose, and cerebrospinal fluid shunting.
- When used concomitantly with cisplatin, radiation therapy can substantially exacerbate the hearing loss associated with platinum chemotherapy.[23,27,28,29]
- In two reports from the CCSS, the following clinical and genetic factors were reported in 5-year cancer survivors:
- Increased risk of problems with hearing sounds (relative risk [RR], 2.3), tinnitus (RR, 1.7), hearing loss requiring an aid (RR, 4.4), and hearing loss in one or both ears not corrected by a hearing aid (RR, 5.2), compared with siblings. Temporal lobe irradiation (>30 Gy) and posterior fossa irradiation (>50 Gy but also 30–49.9 Gy) were associated with these adverse outcomes.[6]
- Exposure to platinum was associated with an increased risk of problems with hearing sounds (RR, 2.1), tinnitus (RR, 2.8), and hearing loss requiring an aid (RR, 4.1).[6]
- Males were more likely than females to report cranial radiation therapy–related tinnitus (9.4% vs. 5.4%) and hearing loss (14.0% vs. 10.7%).[30]
- Survivors with tinnitus or hearing loss were more likely than controls to experience persistent dizziness or vertigo, take antidepressants, and report poorer overall health.[30]
- A genome-wide association study identified a single-nucleotide polymorphism on chromosome 6 as nearly genome-wide significant for association with cranial radiation therapy–related hearing loss. A replication analysis in the St. Jude Life (SJLIFE) cohort study supported its significant association with cranial radiation therapy–related and de novo hearing loss.[30]
- The Pediatric Normal Tissue Effects in the Clinic (PENTEC) investigation included 457 cochleas at risk in patients treated with radiation therapy without chemotherapy, and 398 cochleas in patients treated with chemotherapy.[31]
- The incidence and severity of cochlear hearing loss from radiation therapy exposure alone is related to dose and age. Risk of hearing loss was less than 5% in cochlea receiving a mean dose 35 Gy or lower but increased to 30% at a dose of 50 Gy.
- Hearing loss risk ranged from 25% to 40% in children younger than 5 years at diagnosis, declining to 10% in older children for any radiation dose.
- Probability of similar severe hearing loss occurred at doses 18.3 Gy higher for children younger than 3 years than those older than 3 years.
- High-frequency hearing loss was most common, with average onset occurring 3.6 years (range, 0.4–13.2 years) after radiation therapy.
- Low-frequency hearing loss was also seen after radiation therapy (in contrast with chemotherapy that primarily affects high frequency).
- Chronic comorbidities were assessed in a cohort of 6,778 2-year survivors of adolescent and young adult cancer (defined as diagnosed with cancer between the ages of 15–39 years), with a median follow-up of 5.1 years after cancer diagnosis. The risk of developing chronic comorbidities was compared with matched individuals without a history of cancer. The crude incidence rate ratio (IRR) was 3.0 for hearing loss associated with radiation exposure higher than 30 Gy to the head. In multivariate analyses, a combined exposure to platinum (>450 mg/m2) and radiation to the head (30 Gy) was associated with increased risk of hearing loss (IRR,14.9).[32]
Hearing loss and quality of life
Children treated for malignancies may be at risk of early- or delayed-onset hearing loss. This can affect learning, communication, school performance, social interaction, and overall quality of life.
- Hearing sensitivity is measured by pure-tone audiometry. This assessment does not measure the social and emotional impact of hearing loss. The functional impact of treatment-induced hearing loss on quality of life was examined in adult survivors of childhood cancer from the SJLIFE cohort study. These survivors had been treated with platinum chemotherapy or head/neck radiation therapy and had documented hearing loss (n = 237).[33] Participants were assessed using the Hearing Handicap Inventory for Adults. Survivors with severe hearing loss (n = 156) had increased likelihood of social isolation (OR, 8.76), depression (OR, 9.11), anxiety (OR, 17.57), reduced personal income (OR, 2.82), and less full-time employment (OR, 2.47). Survivors who did not use a recommended hearing aid were twice as likely to have less than full-time employment (OR, 2.26) and reduced personal income (OR, 2.24) than survivors who wore a hearing aid.
- Among 137 childhood survivors of neuroblastoma (aged 8–17 years), hearing loss was associated with reading and math skill problems, as well as higher risk of learning disability and/or special education needs. In addition, hearing loss was associated with poorer school-related quality of life.[34]
- Serial neurocognitive and audiology evaluations were performed on 260 children and young adults with embryonal brain tumors who were enrolled on a treatment protocol that consisted of surgery, risk-adapted cranial spinal irradiation, and chemotherapy. The 64 children with severe sensorineural hearing loss exhibited greater reading difficulties over time compared with the group of children with normal or mild-to-moderate sensorineural hearing loss. Specifically, these children with severe sensorineural hearing loss seemed to struggle most with phonological skills and processing speed, which affect higher level skills such as reading comprehension.[35]
- In a study of adult survivors of pediatric CNS tumors (n = 180) and non-CNS solid tumors (n = 226) who were treated with potentially ototoxic cancer therapy, serious hearing loss (requiring aid or resulting in deafness) was associated with a twofold increased risk of dependent living and unemployment or not graduating from high school.[36]
The Children's Oncology Group has published recommendations for the evaluation and management of hearing loss in survivors of childhood and adolescent cancers to promote early identification of at-risk survivors and timely referral for remedial services.
Table 19 summarizes auditory late effects and the related health screenings.
Table 19. Auditory Late Effectsa
Predisposing Therapy |
Potential Auditory Effects |
Health Screening/Interventions |
FM = frequency modulated. |
a Adapted from theChildren's Oncology Group Long-Term Follow-Up Guidelines for Survivors of Childhood, Adolescent, and Young Adult Cancers. |
Platinum agents (cisplatin, carboplatin); radiation exposing the ear |
Otologic toxic effects; sensorineural hearing loss; tinnitus; vertigo; dehydrated ceruminosis; conductive hearing loss |
History: hearing difficulties, tinnitus, vertigo |
Otoscopic examination |
Audiology evaluation |
Amplification in patients with progressive hearing loss |
Speech and language therapy for children with hearing loss |
Otolaryngology consultation in patients with chronic infection, cerumen impaction, or other anatomical problems exacerbating or contributing to hearing loss |
Educational accommodations (e.g., preferential classroom seating, FM amplification system, etc.) |
Orbital and Optic
Orbital complications are common after radiation therapy for retinoblastoma, head and neck sarcomas, CNS tumors, and after total-body irradiation (TBI).
Retinoblastoma
- Progress has been made in the management of retinoblastoma, with better enucleation implants, intravenous chemoreduction, and intra-arterial chemotherapy in addition to intravitreal chemotherapy, thermotherapy, cryotherapy, and plaque radiation therapy.[37,38] Longer follow-up is needed to assess the impact on vision in patients undergoing these more contemporary treatment modalities.
- Previously, tumors located near the macula and fovea were associated with an increased risk of complications leading to vision loss, although treatment of these tumors with foveal laser ablation has shown promise in preserving vision.[39,40]
- In a study of 61 patients (94 eyes) with a mean follow-up of 51.8 months, a mean lens dose of 7 Gy was associated with a 5-year incidence of cataracts of 20% to 25%.[41]
- In a study of 64 eyes from 42 patients (22 bilateral cases) with salvaged retinoblastoma, after a mean follow-up of 8 years, strabismus was found to be a very common adverse sequela, with 69% of patients having some type of deviation. Exotropia (43%) was more common. Foveal tumor at presentation was found to be the only significant risk factor.[42]
- For survivors of retinoblastoma, a small orbital volume may result from either enucleation or radiation therapy.
- Age younger than 1 year may increase the risk of orbital complications, but this finding is not consistent across studies.[43,44]
- A qualitative analysis of survey responses from 404 survivors of retinoblastoma (mean time since diagnosis, 42 years) who had undergone enucleation identified psychosocial effects related to physical and intrapersonal struggles with appearance and social and relational problems, particularly with teasing and bullying.[45]
The PENTEC ocular task force reviewed dosimetric and clinical factors to quantify the radiation dose dependence of late ocular adverse effects, including retinopathy, optic neuropathy, and cataract, in childhood cancer survivors who received cranial radiation therapy.[46]
- Based on limited data, the model for development of retinopathy suggests a 5% and 50% risk of toxicity at 42 Gy and 62 Gy, respectively.
- The model for development of optic neuropathy suggests a 5% and 50% risk of toxicity at 57 Gy and 64 Gy, respectively.
- The models for cataracts suggest a 5% and 50% risk of self-reported cataract at 12 Gy and higher than 40 Gy, respectively, and a 50% risk of ophthalmologist-diagnosed cataract at 9 Gy (>5% long-term risk at 0 Gy in patients treated with chemotherapy only).
For more information about the treatment of retinoblastoma, see Retinoblastoma Treatment.
Rhabdomyosarcoma
- Survivors of orbital rhabdomyosarcoma are at risk of dry eye, cataract, orbital hypoplasia, ptosis, retinopathy, keratoconjunctivitis, optic neuropathy, lid epithelioma, and impairment of vision after radiation therapy doses of 30 Gy to 65 Gy.[47,48] Despite dosimetric precision of proton therapy, orbital asymmetry is reported to develop after higher than 40 Gy relative biological effectiveness (RBE) to multiple bones of the orbital rim.[49]
- Over the past 3 decades, radiation therapy treatment techniques have evolved. These techniques were examined in a multinational, trans-Atlantic study of head and neck rhabdomyosarcoma survivors in which facial deformity likely occurred as a result of local control. Based on tumor site, there was reduced facial growth in survivors with nonparameningeal primary tumors (P = .002), and parameningeal primary tumors (P < .001), but not for orbital primary tumors (P = .080), when compared with healthy controls. In the four different local therapy strategies used (proton therapy, photon therapy, surgery with radiation therapy, and surgery with brachytherapy), local control and survival were comparable. When comparing radiation therapy with surgery and brachytherapy, orbital patients had more facial deformations.[50]
- The higher radiation therapy dose ranges (>50 Gy) are associated with lid epitheliomas, keratoconjunctivitis, lacrimal duct atrophy, and severe dry eye.
- Retinitis and optic neuropathy may also result from doses of 50 Gy to 65 Gy and even at lower total doses if the individual fraction size is higher than 2 Gy.[51]
- Cataracts are reported after lower doses of 10 Gy to 18 Gy.[47]
For more information about the treatment of rhabdomyosarcoma in children, see Childhood Rhabdomyosarcoma Treatment.
Low-grade optic pathway glioma
Survivors of optic pathway gliomas are also at risk of visual complications, resulting in part from tumor proximity to the optic nerve.
- In a retrospective cohort study of 59 pediatric patients with sporadic optic pathway gliomas diagnosed between 1990 and 2014 (median follow-up, 5.2 years), there was a significant burden of long-term visual impairment.[52]
- More than two-thirds of the patients had evidence of long-term vision loss, more than one-half had severe vision loss in at least one eye, and one-quarter of the patients had severe bilateral vision loss.
- Identified risk factors for poor visual outcome were postchiasmal involvement, younger age, and optic nerve pallor at presentation.
- Longitudinal follow-up (mean, 9 years) of 21 patients with optic pathway gliomas indicated that before treatment, 81% of patients had reduced visual acuity, 81% had optic nerve pallor, and all had reduced visual evoked potentials in one or both eyes.[53]
- Treatment arrested acuity loss for 4 to 5 years.
- Visual acuity was stable or improved in 33% of patients at last follow-up; however, it declined on average. Visual acuity at follow-up was related to tumor volume at initial presentation.
- In a study of 51 children with low-grade gliomas and low-grade glioneural tumors diagnosed within the first year of life, visual acuity was decreased in 27 of 48 patients (56%), 13 (27%) of whom were legally blind. The tumor location (hypothalamic or optic pathway) was significantly associated with decreased visual acuity (P = .002).[54]
- CCSS investigators evaluated the impact of impaired vision on cognitive and psychosocial outcomes among 1,233 adult survivors of childhood low-grade gliomas.[55]
- Some degree of visual impairment was prevalent in 22.5% of patients, and 3.8% of patients were blind in both eyes.
- Survivors who were blind in both eyes were more likely to be unmarried, live dependently, and be unemployed than were survivors with unimpaired vision.
- Bilateral blindness did not impact self-reported cognitive or emotional outcomes. Impaired (with some remaining) vision was not associated with psychological or economic outcomes.
Treatment-specific effects
Survivors of childhood cancer are at increased risk of ocular late effects related to both glucocorticoid and radiation exposure to the eye.
Evidence (cataract development from radiation exposure):
- The CCSS reported the increased risk of developing vision late effects in survivors who were 5 or more years from diagnosis. These late effects included cataracts (RR, 10.8), glaucoma (RR, 2.5), legal blindness (RR, 2.6), double vision (RR, 4.1), and dry eye (RR, 1.9), compared with siblings.[56] In a separate report, the authors provided additional data on the interval from radiation therapy and the radiation dose associated with cataract development.[57] Among 13,902 study participants, 3.5% developed cataracts (41% within 5 years of radiation therapy), with a median time to onset of 9.6 years and a maximum time of 37 years. Lens radiation doses were associated with an increased prevalence: 1.3% if less than 0.5 Gy, 6.1% after 2.5 to 3.49 Gy, and 40.6% after 20 to 60 Gy.
- Higher doses were associated with a shorter time interval to diagnosis. Of the group with cataracts, 31% reported having cataract surgery, supporting the clinical consequences.
- Cytosine arabinoside (OR, 1.5) and doxorubicin (OR, 1.5) were independently associated with cataract development, methotrexate was inversely associated (OR, 0.6), and no positive interaction between the use of corticosteroids and radiation therapy was observed.
- In a cohort of 517 survivors of childhood acute lymphoblastic leukemia (median time from diagnosis, 10.9 years) who were all evaluated by slit lamp examination, the 15-year cumulative incidence of cataract was 4.5%. CNS radiation therapy was the only treatment-related risk factor identified for cataract development, which occurred in 11.1% of irradiated survivors, compared with 2.8% of those who were not irradiated.[58]
Visual acuity decline is rare after radiation therapy for intracranial malignancies in children.
Evidence (visual acuity decline from radiation exposure):
- A retrospective study of 458 children (875 at-risk eyes) treated with proton therapy for intracranial malignancy assessed radiation therapy–related visual decline. Eyes were considered at risk if either the ipsilateral optic nerve or optic chiasm received 30 GyRBE or higher to 0.1 cm3.[59]
- No patients experienced complete vision loss.
- The actuarial 5-year rate of visual acuity decline was 2.6%.
- Tumor location significantly correlated with risk. Investigators found that children with tumors of the suprasellar or the optic pathway region are at greatest risk. The reported risk was about 5% decline in visual acuity with a dose of 56 GyRBE to the optic nerve or optic pathway.
- For children with tumors arising elsewhere in the brain, no toxicity was observed.
Chronic comorbidities were assessed in a cohort of 6,778 2-year survivors of adolescent and young adult cancer (defined as diagnosed with cancer between the ages of 15–39 years), with a median follow-up of 5.1 years after cancer diagnosis. The risk of developing chronic comorbidities was compared with matched individuals without a history of cancer. The crude IRR was 8.1 for vision loss associated with radiation exposure higher than 30 Gy to the head.[32]
Ocular complications, such as cataracts and dry eye syndrome, are common after HSCT in childhood.
Evidence (ocular effects of HSCT):
- A St. Jude Children's Research Hospital investigation evaluated ocular complications in school-aged children and adolescents who survived at least 1 year following allogeneic HSCT. Among 162 patients (mean age at transplant, 13.4 years; mean follow-up, 4 years), the following was reported:[60]
- Cataract formation was noted in 57 patients (35%) and dry eye was noted in 51 patients (31%).
- Multivariate analysis of significant variables showed that TBI was a risk factor for cataract formation, and chronic graft-versus-host disease (GVHD) was a significant risk factor for dry eye syndrome.
- Compared with patients treated with busulfan or other chemotherapy, patients treated with single-dose or fractionated TBI are at increased risk of cataracts.[61,62,63,64]
- The risk ranges from approximately 10% to 60% at 10 years posttreatment, depending on the total dose and fractionation, with a shorter latency period and more severe cataracts noted after single fraction and higher dose or dose-rate TBI.
- One series evaluated 48 patients with high-risk neuroblastoma (median years since diagnosis, 14.1 years) who were all treated with multimodal therapy, including high-dose chemotherapy, HSCT (with or without TBI), radiation therapy, and immunotherapy.[10]
- Twenty-five of the survivors (52%) developed cataracts.
- All patients with cataracts had received TBI (P < .0001).
- Patients receiving TBI doses of less than 40 Gy have a less than 10% chance of developing severe cataracts.[64]
- Corticosteroids and GVHD may further increase risk.[61,65]
- The prevalence of cataracts, evaluated by serial slit lamp testing, among 271 participants (mean follow-up, 10.3 years) in the Leucémie de l'Enfant et de l'Adolescent (LEA) program was 41.7%, with 8.1% requiring surgical intervention.[66]
- The cumulative incidence of cataracts among those treated with TBI increased over time from 30% at 5 years to 70.8% at 15 years and 78% at 20 years.
- The lack of a plateau in cataract incidence suggests that nearly all patients treated with TBI will develop cataracts as follow-up increases.
- In contrast, the 15-year cumulative incidence of cataracts was 12.5% among those conditioned with busulfan.
- Multivariable analysis identified high cumulative steroid dose as a potential cofactor with TBI for cataract risk.
- Dry eye syndrome is the most common manifestation of chronic ocular GVHD, but it may occur as an isolated phenomenon in the absence of GVHD.
- A single-center study evaluated 484 consecutive children receiving HSCT who had ophthalmological examinations performed before HSCT and then annually until 5 years after HSCT.[67]
- The cumulate incidence of chronic ocular GVHD was 6.0%.
- The cumulative incidence of new-onset dry eye disease was 8.7%.
- Younger age (0–4 years vs. age 10–16 years) was associated with reduced risk of dry eye disease.
Table 20 summarizes ocular late effects and the related health screenings.
Table 20. Ocular Late Effectsa
Predisposing Therapy |
Ocular/Vision Effects |
Health Screening/Interventions |
GVHD = graft-versus-host disease; HSCT = hematopoietic stem cell transplant; 131I = iodine I 131. |
a Adapted from theChildren's Oncology Group Long-Term Follow-Up Guidelines for Survivors of Childhood, Adolescent, and Young Adult Cancers. |
Busulfan; corticosteroids; radiation exposing the eye |
Cataracts |
History: decreased acuity, halos, diplopia |
Eye examination: visual acuity, funduscopy (yearly) |
Ophthalmology consultation |
Radiation exposing the eye, including radioiodine (131I) |
Ocular toxicity (orbital hypoplasia, lacrimal duct atrophy, xerophthalmia [keratoconjunctivitis sicca], keratitis, telangiectasias, retinopathy, optic chiasm neuropathy, enophthalmos, chronic painful eye, maculopathy, papillopathy, glaucoma) |
History: visual changes (decreased acuity, halos, diplopia), dry eye, persistent eye irritation, excessive tearing, light sensitivity, poor night vision, painful eye |
Eye examination: visual acuity, funduscopy (yearly) |
Ophthalmology consultation |
HSCT with any history of chronic GVHD |
Xerophthalmia (keratoconjunctivitis sicca) |
History: dry eye (burning, itching, foreign body sensation, inflammation) |
Eye examination: visual acuity, funduscopy (yearly) |
Enucleation |
Impaired cosmesis; poor prosthetic fit; orbital hypoplasia |
Ocular prosthetic evaluation |
Ophthalmology |
For information on the late effects of special senses, including risk factors, evaluation, and health counseling, see the Children's Oncology Group Long-Term Follow-Up Guidelines for Survivors of Childhood, Adolescent, and Young Adult Cancers.
References:
-
Li Y, Womer RB, Silber JH: Predicting cisplatin ototoxicity in children: the influence of age and the cumulative dose. Eur J Cancer 40 (16): 2445-51, 2004.
-
Keilty D, Khandwala M, Liu ZA, et al.: Hearing Loss After Radiation and Chemotherapy for CNS and Head-and-Neck Tumors in Children. J Clin Oncol 39 (34): 3813-3821, 2021.
-
Weiss A, Sommer G, Kasteler R, et al.: Long-term auditory complications after childhood cancer: A report from the Swiss Childhood Cancer Survivor Study. Pediatr Blood Cancer 64 (2): 364-373, 2017.
-
Strebel S, Mader L, Sláma T, et al.: Severity of hearing loss after platinum chemotherapy in childhood cancer survivors. Pediatr Blood Cancer 69 (9): e29755, 2022.
-
Beyea JA, Lau C, Cooke B, et al.: Long-Term Incidence and Predictors of Significant Hearing Loss Requiring Hearing Assistive Devices Among Childhood Cancer Survivors: A Population-Based Study. J Clin Oncol 38 (23): 2639-2646, 2020.
-
Whelan K, Stratton K, Kawashima T, et al.: Auditory complications in childhood cancer survivors: a report from the childhood cancer survivor study. Pediatr Blood Cancer 57 (1): 126-34, 2011.
-
Moke DJ, Luo C, Millstein J, et al.: Prevalence and risk factors for cisplatin-induced hearing loss in children, adolescents, and young adults: a multi-institutional North American cohort study. Lancet Child Adolesc Health 5 (4): 274-283, 2021.
-
Clemens E, de Vries AC, Pluijm SF, et al.: Determinants of ototoxicity in 451 platinum-treated Dutch survivors of childhood cancer: A DCOG late-effects study. Eur J Cancer 69: 77-85, 2016.
-
Brock PR, Knight KR, Freyer DR, et al.: Platinum-induced ototoxicity in children: a consensus review on mechanisms, predisposition, and protection, including a new International Society of Pediatric Oncology Boston ototoxicity scale. J Clin Oncol 30 (19): 2408-17, 2012.
-
Hobbie WL, Li Y, Carlson C, et al.: Late effects in survivors of high-risk neuroblastoma following stem cell transplant with and without total body irradiation. Pediatr Blood Cancer 69 (3): e29537, 2022.
-
Kushner BH, Budnick A, Kramer K, et al.: Ototoxicity from high-dose use of platinum compounds in patients with neuroblastoma. Cancer 107 (2): 417-22, 2006.
-
Landier W, Knight K, Wong FL, et al.: Ototoxicity in children with high-risk neuroblastoma: prevalence, risk factors, and concordance of grading scales--a report from the Children's Oncology Group. J Clin Oncol 32 (6): 527-34, 2014.
-
Lewis MJ, DuBois SG, Fligor B, et al.: Ototoxicity in children treated for osteosarcoma. Pediatr Blood Cancer 52 (3): 387-91, 2009.
-
Freyer DR, Chen L, Krailo MD, et al.: Effects of sodium thiosulfate versus observation on development of cisplatin-induced hearing loss in children with cancer (ACCL0431): a multicentre, randomised, controlled, open-label, phase 3 trial. Lancet Oncol 18 (1): 63-74, 2017.
-
Brock PR, Maibach R, Childs M, et al.: Sodium Thiosulfate for Protection from Cisplatin-Induced Hearing Loss. N Engl J Med 378 (25): 2376-2385, 2018.
-
Orgel E, Knight KR, Villaluna D, et al.: Reevaluation of sodium thiosulfate otoprotection using the consensus International Society of Paediatric Oncology Ototoxicity Scale: A report from the Children's Oncology Group study ACCL0431. Pediatr Blood Cancer : e30550, 2023.
-
Clemens E, de Vries AC, Am Zehnhoff-Dinnesen A, et al.: Hearing loss after platinum treatment is irreversible in noncranial irradiated childhood cancer survivors. Pediatr Hematol Oncol 34 (2): 120-129, 2017.
-
Kolinsky DC, Hayashi SS, Karzon R, et al.: Late onset hearing loss: a significant complication of cancer survivors treated with Cisplatin containing chemotherapy regimens. J Pediatr Hematol Oncol 32 (2): 119-23, 2010.
-
Fouladi M, Gururangan S, Moghrabi A, et al.: Carboplatin-based primary chemotherapy for infants and young children with CNS tumors. Cancer 115 (14): 3243-53, 2009.
-
Jehanne M, Lumbroso-Le Rouic L, Savignoni A, et al.: Analysis of ototoxicity in young children receiving carboplatin in the context of conservative management of unilateral or bilateral retinoblastoma. Pediatr Blood Cancer 52 (5): 637-43, 2009.
-
Qaddoumi I, Bass JK, Wu J, et al.: Carboplatin-associated ototoxicity in children with retinoblastoma. J Clin Oncol 30 (10): 1034-41, 2012.
-
Funt SA, Knezevic A, Wilson K, et al.: Ototoxicity associated with high-dose carboplatin for patients with previously treated germ cell tumors. Cancer 129 (24): 3952-3961, 2023.
-
Hua C, Bass JK, Khan R, et al.: Hearing loss after radiotherapy for pediatric brain tumors: effect of cochlear dose. Int J Radiat Oncol Biol Phys 72 (3): 892-9, 2008.
-
Bhandare N, Jackson A, Eisbruch A, et al.: Radiation therapy and hearing loss. Int J Radiat Oncol Biol Phys 76 (3 Suppl): S50-7, 2010.
-
Merchant TE, Gould CJ, Xiong X, et al.: Early neuro-otologic effects of three-dimensional irradiation in children with primary brain tumors. Int J Radiat Oncol Biol Phys 58 (4): 1194-207, 2004.
-
Bass JK, Hua CH, Huang J, et al.: Hearing Loss in Patients Who Received Cranial Radiation Therapy for Childhood Cancer. J Clin Oncol 34 (11): 1248-55, 2016.
-
Cheuk DK, Billups CA, Martin MG, et al.: Prognostic factors and long-term outcomes of childhood nasopharyngeal carcinoma. Cancer 117 (1): 197-206, 2011.
-
Merchant TE, Hua CH, Shukla H, et al.: Proton versus photon radiotherapy for common pediatric brain tumors: comparison of models of dose characteristics and their relationship to cognitive function. Pediatr Blood Cancer 51 (1): 110-7, 2008.
-
Paulino AC, Lobo M, Teh BS, et al.: Ototoxicity after intensity-modulated radiation therapy and cisplatin-based chemotherapy in children with medulloblastoma. Int J Radiat Oncol Biol Phys 78 (5): 1445-50, 2010.
-
Trendowski MR, Baedke JL, Sapkota Y, et al.: Clinical and genetic risk factors for radiation-associated ototoxicity: A report from the Childhood Cancer Survivor Study and the St. Jude Lifetime Cohort. Cancer 127 (21): 4091-4102, 2021.
-
Murphy B, Jackson A, Bass JK, et al.: Modeling the Risk of Hearing Loss From Radiation Therapy in Childhood Cancer Survivors: A PENTEC Comprehensive Review. Int J Radiat Oncol Biol Phys 119 (2): 446-456, 2024.
-
Chao C, Bhatia S, Xu L, et al.: Chronic Comorbidities Among Survivors of Adolescent and Young Adult Cancer. J Clin Oncol 38 (27): 3161-3174, 2020.
-
Bass JK, Wang F, Thaxton ME, et al.: Association of hearing loss with patient-reported functional outcomes in adult survivors of childhood cancer. J Natl Cancer Inst 116 (4): 596-605, 2024.
-
Gurney JG, Tersak JM, Ness KK, et al.: Hearing loss, quality of life, and academic problems in long-term neuroblastoma survivors: a report from the Children's Oncology Group. Pediatrics 120 (5): e1229-36, 2007.
-
Olivier TW, Bass JK, Ashford JM, et al.: Cognitive Implications of Ototoxicity in Pediatric Patients With Embryonal Brain Tumors. J Clin Oncol 37 (18): 1566-1575, 2019.
-
Brinkman TM, Bass JK, Li Z, et al.: Treatment-induced hearing loss and adult social outcomes in survivors of childhood CNS and non-CNS solid tumors: Results from the St. Jude Lifetime Cohort Study. Cancer 121 (22): 4053-61, 2015.
-
Shields CL, Bas Z, Tadepalli S, et al.: Long-term (20-year) real-world outcomes of intravenous chemotherapy (chemoreduction) for retinoblastoma in 964 eyes of 554 patients at a single centre. Br J Ophthalmol 104 (11): 1548-1555, 2020.
-
Francis JH, Brodie SE, Marr B, et al.: Efficacy and Toxicity of Intravitreous Chemotherapy for Retinoblastoma: Four-Year Experience. Ophthalmology 124 (4): 488-495, 2017.
-
Shields CL, Shields JA, Cater J, et al.: Plaque radiotherapy for retinoblastoma: long-term tumor control and treatment complications in 208 tumors. Ophthalmology 108 (11): 2116-21, 2001.
-
Schefler AC, Cicciarelli N, Feuer W, et al.: Macular retinoblastoma: evaluation of tumor control, local complications, and visual outcomes for eyes treated with chemotherapy and repetitive foveal laser ablation. Ophthalmology 114 (1): 162-9, 2007.
-
Nguyen SM, Sison J, Jones M, et al.: Lens Dose-Response Prediction Modeling and Cataract Incidence in Patients With Retinoblastoma After Lens-Sparing or Whole-Eye Radiation Therapy. Int J Radiat Oncol Biol Phys 103 (5): 1143-1150, 2019.
-
Fabian ID, Stacey AW, Naeem Z, et al.: Strabismus in retinoblastoma survivors with long-term follow-up. J AAPOS 22 (4): 276.e1-276.e7, 2018.
-
Kaste SC, Chen G, Fontanesi J, et al.: Orbital development in long-term survivors of retinoblastoma. J Clin Oncol 15 (3): 1183-9, 1997.
-
Peylan-Ramu N, Bin-Nun A, Skleir-Levy M, et al.: Orbital growth retardation in retinoblastoma survivors: work in progress. Med Pediatr Oncol 37 (5): 465-70, 2001.
-
Banerjee SC, Pottenger E, Petriccione M, et al.: Impact of enucleation on adult retinoblastoma survivors' quality of life: A qualitative study of survivors' perspectives. Palliat Support Care 18 (3): 322-331, 2020.
-
Shen CJ, Kry SF, Buchsbaum JC, et al.: Retinopathy, Optic Neuropathy, and Cataract in Childhood Cancer Survivors Treated With Radiation Therapy: A PENTEC Comprehensive Review. Int J Radiat Oncol Biol Phys 119 (2): 431-445, 2024.
-
Raney RB, Anderson JR, Kollath J, et al.: Late effects of therapy in 94 patients with localized rhabdomyosarcoma of the orbit: Report from the Intergroup Rhabdomyosarcoma Study (IRS)-III, 1984-1991. Med Pediatr Oncol 34 (6): 413-20, 2000.
-
Paulino AC, Simon JH, Zhen W, et al.: Long-term effects in children treated with radiotherapy for head and neck rhabdomyosarcoma. Int J Radiat Oncol Biol Phys 48 (5): 1489-95, 2000.
-
Hol MLF, Indelicato DJ, Rotondo RL, et al.: Dose-Effect Analysis of Early Changes in Orbital Bone Morphology After Radiation Therapy for Rhabdomyosarcoma. Pract Radiat Oncol 10 (1): 53-58, 2020.
-
Hol MLF, Indelicato DJ, Slater O, et al.: Facial deformation following treatment for pediatric head and neck rhabdomyosarcoma; the difference between treatment modalities. Results of a trans-Atlantic, multicenter cross-sectional cohort study. Pediatr Blood Cancer 70 (8): e30412, 2023.
-
Kline LB, Kim JY, Ceballos R: Radiation optic neuropathy. Ophthalmology 92 (8): 1118-26, 1985.
-
Wan MJ, Ullrich NJ, Manley PE, et al.: Long-term visual outcomes of optic pathway gliomas in pediatric patients without neurofibromatosis type 1. J Neurooncol 129 (1): 173-8, 2016.
-
Kelly JP, Leary S, Khanna P, et al.: Longitudinal measures of visual function, tumor volume, and prediction of visual outcomes after treatment of optic pathway gliomas. Ophthalmology 119 (6): 1231-7, 2012.
-
Liu APY, Hastings C, Wu S, et al.: Treatment burden and long-term health deficits of patients with low-grade gliomas or glioneuronal tumors diagnosed during the first year of life. Cancer 125 (7): 1163-1175, 2019.
-
de Blank PM, Fisher MJ, Lu L, et al.: Impact of vision loss among survivors of childhood central nervous system astroglial tumors. Cancer 122 (5): 730-9, 2016.
-
Whelan KF, Stratton K, Kawashima T, et al.: Ocular late effects in childhood and adolescent cancer survivors: a report from the childhood cancer survivor study. Pediatr Blood Cancer 54 (1): 103-9, 2010.
-
Chodick G, Sigurdson AJ, Kleinerman RA, et al.: The Risk of Cataract among Survivors of Childhood and Adolescent Cancer: A Report from the Childhood Cancer Survivor Study. Radiat Res 185 (4): 366-74, 2016.
-
Alloin AL, Barlogis V, Auquier P, et al.: Prevalence and risk factors of cataract after chemotherapy with or without central nervous system irradiation for childhood acute lymphoblastic leukaemia: an LEA study. Br J Haematol 164 (1): 94-100, 2014.
-
Bates JE, Indelicato DJ, Morris CG, et al.: Visual decline in pediatric survivors of brain tumors following radiotherapy. Acta Oncol 59 (10): 1257-1262, 2020.
-
Hoehn ME, Vestal R, Calderwood J, et al.: Ocular Complications in School-Age Children and Adolescents after Allogeneic Bone Marrow Transplantation. Am J Ophthalmol 213: 153-160, 2020.
-
Ferry C, Gemayel G, Rocha V, et al.: Long-term outcomes after allogeneic stem cell transplantation for children with hematological malignancies. Bone Marrow Transplant 40 (3): 219-24, 2007.
-
Fahnehjelm KT, Törnquist AL, Olsson M, et al.: Visual outcome and cataract development after allogeneic stem-cell transplantation in children. Acta Ophthalmol Scand 85 (7): 724-33, 2007.
-
Gurney JG, Ness KK, Rosenthal J, et al.: Visual, auditory, sensory, and motor impairments in long-term survivors of hematopoietic stem cell transplantation performed in childhood: results from the Bone Marrow Transplant Survivor study. Cancer 106 (6): 1402-8, 2006.
-
Kal HB, VAN Kempen-Harteveld ML: Induction of severe cataract and late renal dysfunction following total body irradiation: dose-effect relationships. Anticancer Res 29 (8): 3305-9, 2009.
-
Holmström G, Borgström B, Calissendorff B: Cataract in children after bone marrow transplantation: relation to conditioning regimen. Acta Ophthalmol Scand 80 (2): 211-5, 2002.
-
Horwitz M, Auquier P, Barlogis V, et al.: Incidence and risk factors for cataract after haematopoietic stem cell transplantation for childhood leukaemia: an LEA study. Br J Haematol 168 (4): 518-25, 2015.
-
Jeppesen H, Kielsen K, Siersma V, et al.: Ocular graft-versus-host disease and dry eye disease after paediatric haematopoietic stem cell transplantation - incidence and risk factors. Bone Marrow Transplant 57 (3): 487-498, 2022.
Late Effects of the Urinary System
Acute toxicity of the urinary system from cancer therapy is well known. Less is known about the genitourinary outcomes in long-term survivors.[1] The evidence for long-term renal injury in childhood cancer survivors is limited by studies characterized by small sample size, cohort selection and participation bias, cross-sectional assessment, heterogeneity in time since treatment, and method of ascertainment. In particular, the inaccuracies of diagnosing chronic kidney dysfunction by estimating equations of glomerular dysfunction should be considered.[2]
The incidence of late major surgical interventions among childhood cancer survivors was examined through data from the Childhood Cancer Survivor Study (CCSS). Childhood cancer survivors were more likely to undergo a major renal or urinary surgical late intervention than siblings (adjusted relative risk [RR], 2.0). Locoregional surgery or radiation therapy cancer treatments were associated with undergoing late surgical intervention in the same body region or organ system.[3]
Cancer treatments predisposing to renal injury and/or high blood pressure later in life include the following:
- Chemotherapeutic drugs (cisplatin, carboplatin, ifosfamide, methotrexate).
- Renal radiation therapy.
- Nephrectomy.
The risk and degree of renal dysfunction depend on type and intensity of therapy, and the interpretation of the studies is compromised by variability in testing.
The incidence of and risk factors for late-onset kidney failure among long-term survivors of childhood cancer has not been well studied.
Among 25,530 CCSS participants (median follow-up, 22.3 years), the 35-year cumulative incidence of self-reported late-onset kidney failure—defined as dialysis, renal transplant, or death attributable to kidney disease—was 1.7% (compared with 0.2% in the sibling cohort).[4]
- Independent treatment risk factors for late-onset kidney failure included high-dose (>15 Gy) radiation exposing the kidney (RR, 4.0), high-dose (>250 mg/m2) anthracycline (RR, 1.6), any ifosfamide dose (RR, 2.6), and nephrectomy (RR, 1.9).
- Modifiable risk factors associated with late-onset kidney failure included hypertension (RR, 14.4 with history of nephrectomy; RR, 5.9 without history of nephrectomy) and diabetes (RR, 2.2).
Few large-scale studies have evaluated late renal-health outcomes and risk factors for renal dysfunction among survivors treated with potentially nephrotoxic modalities.
Evidence (renal dysfunction in childhood cancer survivors):
- The Dutch Childhood Cancer Survivor Study (DCCSS)-LATER 2 kidney analysis, a nationwide cross-sectional cohort study, evaluated 1,024 cancer survivors (aged 18 years or older at the time of the study). These patients were 5 or more years from diagnosis and treated between 1963 and 2001 with nephrectomy, abdominal radiation therapy, total-body irradiation (TBI), cisplatin, carboplatin, ifosfamide, high-dose cyclophosphamide, or hematopoietic stem cell transplant (HSCT). These survivors were compared with 500 age- and sex-matched controls.[5]
- In 3.7% of the survivors (median age, 32 years), the glomerular filtration rate (GFR) was under 60 mL/min/1.73m2, compared with none of the controls showing a GFR decrease.
- End-stage kidney disease was present in 1.1% of survivors.
- Chronic kidney disease, according to age-thresholds (GFR under 75 for people aged <40 years, GFR under 60 for people aged 40–65 years, and GFR under 40 for people aged >65 years), was 6.6% in survivors, compared with 0.2% in controls.
- Risk factors for chronic kidney disease, based on multivariable analyses, included treatment with nephrectomy (odds ratio [OR], 3.7), abdominal radiation therapy (OR, 1.8), ifosfamide (especially >12 gm/m2; OR, 2.9) and cisplatin doses higher than 500 mg/m2 (OR, 7.2).
- Albuminuria (albumin-to-creatinine-ratio over 3 mg/mmol) was identified in 16.2% of survivors and 1.2% of controls. Risk factors for albuminuria included treatment with TBI (OR, 2.3), abdominal radiation therapy higher than 30 Gy (OR, 2.6), and ifosfamide (OR, 1.6).
- After a median follow-up of 25.5 years, the overall prevalence of electrolyte losses in childhood cancer survivors (magnesium 5.6%, potassium 4.5%, phosphate 5.5%) was not higher than that in controls.[6]
- Risk factors for hypertension were abdominal radiation therapy and TBI. Hypertension is associated with decreased GFR in childhood cancer survivors.[6]
- French CCSS investigators linked national health care system data with clinical and treatment data from medical records to evaluate long-term risk of renal- or urinary-related hospitalization in survivors of childhood cancer.[7]
- Among 5,498 survivors (median age at diagnosis, 5 years; median time from diagnosis, 22.9 years), 230 were hospitalized for renal or urinary tract indications.
- The most common indication for renal hospitalization was dialysis (64.5%), whereas indications for urinary-related hospitalization included urolithiasis (73.2%), followed by diseases of the bladder (15.7%).
- The risk of renal hospitalization was almost threefold (standardized hospitalization ratio, 2.9; 95% confidence interval [CI], 2.4–3.4) compared with the general population.
- Exposing more than 10% of the kidney volume to at least 20 Gy of radiation was associated with a twofold (standardized hospitalization ratio, 2.2; 95% CI, 1.3–3.6) increased risk of hospitalization for renal causes.
- Survivors treated with nephrectomy and high doses of ifosfamide (>60 g/m2) had more than a 20-fold risk of hospitalization for renal causes (RR, 23.7; 95% CI, 5.1–110.0).
Chronic comorbidities were assessed in a cohort of 6,778 2-year survivors of adolescent and young adult (AYA) cancer (defined as diagnosed with cancer between ages 15–39 years), with a median follow-up of 5.1 years after cancer diagnosis. Survivors of AYA cancer had a twofold to threefold increased risk of renal failure. The risk of developing chronic comorbidities was compared with matched individuals without a history of cancer.[8]
Therapy-Related Factors Affecting the Kidney
Cancer treatments predisposing to late renal injury and hypertension include the following:[9,10]
Nephrectomy
- Survivors of childhood cancer who have undergone nephrectomy are at risk of hyperfiltration injury.
- Compensatory hypertrophy of the remaining kidney typically occurs after nephrectomy, but over time, renal injury may manifest as reduced glomerular filtration, microalbuminuria and proteinuria, hypertension, and, rarely, focal glomerulosclerosis leading to chronic renal failure.[9,11]
- Patients with nonsyndromic unilateral Wilms tumor treated with unilateral radical nephrectomy have a 35-year cumulative incidence of late kidney failure of 2.4%, an approximately 10-fold increased rate relative to siblings. No single chemotherapy–related or radiation therapy–related exposure was associated with late kidney failure in a CCSS study of late health outcomes in Wilms tumor survivors (n = 2,008).[12]
- A meta-analysis examined data from 23 studies of patients with Wilms tumor (293 unilateral cases and 386 bilateral cases). The study demonstrated significantly increased odds of developing abnormal kidney function (OR, 4.29; 95% CI, 1.02–18.00) and having a lower estimated GFR (mean, -8.99) among patients treated with radical nephrectomy, compared with those treated with nephron-sparing surgery. Patients with bilateral Wilms tumor (mean follow-up, 7.7 years) treated with radical nephrectomy and contralateral nephron-sparing surgery had higher odds of developing abnormal kidney function (OR, 3.82; 95% CI, 1.76–8.33) and hypertension (OR, 5.81; 95% CI, 1.31–25.86), compared with those treated with bilateral nephron-sparing surgery.[13]
Chemotherapy
Cisplatin, carboplatin, and ifosfamide are all risk factors for the development of kidney tubular dysfunction.[5]
Cisplatin
Cisplatin can cause glomerular and tubular damage, resulting in a diminished GFR and electrolyte wasting (particularly magnesium, calcium, and potassium).
- Acute cisplatin-related nephrotoxicity has been reported in 30% to 100% of exposed children.[14] However, the prevalence of persistent renal dysfunction in long-term survivors appears to be considerably lower.
- The DCCSS-LATER 2 kidney analysis, a nationwide cross-sectional cohort study, evaluated 1,024 survivors (aged 18 years or older at the time of the study) for long-term tubular dysfunction. These patients were 5 or more years from diagnosis and treated between 1963 and 2001 with nephrectomy, abdominal radiation therapy, TBI, cisplatin, carboplatin, ifosfamide, high-dose cyclophosphamide, or HSCT. Survivors were compared with 500 age- and sex-matched controls.[6]
- In general, prevalence and ORs for magnesium loss were significantly increased for childhood cancer survivors treated with cisplatin only (25.8%) or in combination with carboplatin (23.1%).
- Magnesium loss was associated with all doses of cisplatin but highest for a cumulative dose greater than 500 mg/m2 (OR, 22.0). Childhood cancer survivors exposed only to cisplatin were at risk for potassium loss in comparison with controls (prevalence, 12%; OR, 3.2).
- At cumulative doses of cisplatin of higher than 500 mg/m2, the odds for potassium (OR, 17.7) and phosphate loss (OR, 3.6) were increased.
- Among 63 children treated with platinum agents, GFR was less than 60 mL/min per 1.73 m2 in 11% of children, and hypomagnesemia requiring oral supplements occurred in 7% of children at 10 years from completion of therapy.[15]
Carboplatin
Carboplatin is a cisplatin analogue and is less nephrotoxic than cisplatin.
- In a prospective, longitudinal, single-center, cohort study of children monitored for more than 10 years after cisplatin or carboplatin therapy, older age at treatment was found to be the major risk factor for nephrotoxicity, especially for patients who received carboplatin, while cisplatin dose schedule and cumulative carboplatin dose were also important predictors of toxicity. Platinum nephrotoxicity did not change significantly over 10 years.[15]
- The DCCSS-LATER 2 kidney analysis, a nationwide cross-sectional cohort study, evaluated 1,024 survivors (aged 18 years or older at the time of the study) for long-term tubular dysfunction. In multivariable risk analysis, after a median follow-up of 25.5 years, carboplatin was associated with potassium loss at a cumulative dose higher than 2,800 mg/m2 (OR, 5.1).[6]
- The combination of carboplatin and ifosfamide may be associated with more renal damage than the combination of cisplatin and ifosfamide.[15,16,17]
Ifosfamide
Ifosfamide can also cause glomerular and tubular toxicity, with renal tubular acidosis and Fanconi syndrome, a proximal tubular defect characterized by impairment of resorption of glucose, amino acids, phosphate, and bicarbonate.
- The DCCSS-LATER 2 kidney analysis, a nationwide cross-sectional cohort study, evaluated 1,024 survivors (aged 18 years or older at the time of the study) who had received potentially nephrotoxic therapy for long-term tubular dysfunction. In multivariable risk analysis, after a median follow-up of 25.5 years, ifosfamide was associated with potassium loss (OR, 2.4) and phosphate loss (OR, 2.3) (with cumulative dose >42 g/m2).[6]
- Ifosfamide doses greater than 60 g/m2, age younger than 5 years at treatment, and combination with cisplatin and carboplatin increase the risk of ifosfamide-associated renal tubular toxicity.[18,19,20]
Methotrexate
High-dose methotrexate (1,000–33,000 mg/m2) has been reported to cause acute renal dysfunction in up to 12.4% of patients. Long-term renal sequelae have not been described.[21]
Radiation therapy
Radiation therapy to the kidney can result in radiation nephritis or nephropathy after a latent period of 3 to 12 months.
- The kidney is relatively radiosensitive, with a tolerance dose of 20 Gy.[22]
- Doses of 18 Gy are considered unlikely to cause severe or chronic renal sequelae. In contrast, up to 50% of individuals treated with 20 Gy may develop glomerular dysfunction or hypertension within 20 years.[23]
- Specific quantitative data are sparse, but a study of 108 children treated for Wilms tumor who had undergone unilateral nephrectomy showed that 41% of children who received less than 12 Gy of radiation to the contralateral remaining kidney, 56% of children who received 12 Gy to 24 Gy, and 91% of children who received more than 24 Gy had a decreased creatinine clearance (GFR <63 mL/min/m2).[24]
- In a report from the German Registry for the Evaluation of Side Effects after Radiation in Childhood and Adolescence (RISK consortium), 126 patients who underwent radiation therapy to parts of the kidneys for various cancers were evaluated. All patients had also received potentially nephrotoxic chemotherapy. Whole-kidney volumes exposed to greater than 20 Gy (P = .031) or 30 Gy (P = .003) of radiation were associated with a greater risk of nephrotoxicity.[25]
- Risk factors for radiation injury include the following:
- Age at time of radiation therapy.
- Neonates appear to have an increased sensitivity to radiation therapy; doses of 12 Gy to 24 Gy at 1.25 Gy to 1.5 Gy per fraction to the entire kidney were associated with a decreased GFR.[26]
- However, for older children, there is no convincing evidence that age at the time of radiation therapy is related to renal injury.[26]
- Unilateral versus bilateral radiation therapy.
- In the National Wilms Tumor Study experience, renal failure was more common in children with bilateral tumors than in children with unilateral tumors.[27]
- The effects of radiation also depend on whether partial or whole-kidney radiation therapy is administered. Renal failure is rare after the administration of partial-volume radiation doses between 12 Gy and 27 Gy.[28]
- When certain agents such as cyclosporine and teniposide are not used, TBI doses of up to 13 Gy are associated with a less than 8% incidence of kidney toxicity.[29]
- HSCT.
- Chronic kidney disease is a long-term complication of HSCT that has been variably associated with acute kidney injury, lower pretransplant renal function, TBI, conditioning regimens such as fludarabine, graft-versus-host disease, and use of calcineurin inhibitors.[30,31,32]
- Most reports of renal outcomes among long-term survivors of childhood cancer treated with HSCT are limited to descriptive outcomes of very small cohorts.
- For more information, see the Urinary System Late Effects section in Complications, Graft-Versus-Host Disease, and Late Effects After Pediatric Hematopoietic Stem Cell Transplant.
A comprehensive Pediatric Normal Tissue Effects in the Clinic (PENTEC) review by the genitourinary task force evaluated dosimetric and clinical factors to radiation dose-volume relationships for genitourinary dysfunction, including kidney, bladder, and hypertension, for pediatric malignancies. The effect of chemotherapy was also considered.[33]
- The risk of toxicity from 10.5 Gy whole-abdomen irradiation was low among patients with Wilms tumor (risks of ≥grade 2 toxicity, 4% and risks of ≥grade 3 toxicity, 1%).
- For fractionated 12-Gy TBI with various combinations of chemotherapy, the risk of severe toxicity was low, but low-grade toxicity was more common (risks of ≥grade 2 toxicity, 8% and risks of ≥grade 3 toxicity, 3%).
- With a cisplatin dose of 480 mg/m2, a 3% risk of grade 3 or higher toxicity occurred without radiation, and a 5% risk when 26% of the kidney received 10 Gy or higher.
- With an ifosfamide dose of 63 g/m2, a 5% risk of grade 3 or higher toxicity occurred without radiation, and a 10% toxicity risk occurred when 42% of the kidney received 10 Gy or higher.
Renal Function in Survivors of Nonsyndromic Wilms Tumor
- Researchers used the CCSS to evaluate the long-term mortality and chronic health conditions among unilateral, nonsyndromic Wilms tumor survivors (n = 2,008) diagnosed over 3 decades. The 35-year cumulative incidence of late kidney failure among survivors was 2.4% (approximately 10-fold increased rate relative to siblings). The 20-year rate for late kidney failure was 1.7%, and it increased with age. Survivors were at increased risk for kidney failure (RR, 11.9), compared with siblings.[12]
- St. Jude Life (SJLIFE) study investigators evaluated the impact of specific treatment modalities on long-term renal function and blood pressure among adult survivors of Wilms tumor.[34]
- Among 40 survivors of unilateral nonsyndromic Wilms tumor, the prevalence of hypertension was significantly increased for unirradiated (25%) and irradiated (35%) survivors, compared with the prevalence in 35 noncancer controls (0%).
- No irradiated or unirradiated participant had an estimated GFR (creatinine + cystatin C) less than 60 mL/min/1.73 m2.
Genetic Factors Predisposing to Renal Dysfunction
- Many childhood survivors of Wilms tumor who develop chronic renal failure have syndromes accompanying WT1 variants or deletions that predispose to renal disease.
- Data from the National Wilms Tumor Study Group and the U.S. Renal Data System indicate that the 20-year cumulative incidence of end-stage renal disease is 74% in children with unilateral Wilms tumor and Denys-Drash syndrome, 36% for those with WAGR (Wilms tumor, aniridia, genitourinary abnormalities, and range of developmental delays) syndrome, 7% for male patients with genitourinary anomalies, and 0.6% for patients with none of these conditions.[35]
- For patients with bilateral Wilms tumors, the incidence of end-stage renal disease is 50% for Denys-Drash syndrome, 90% for WAGR syndrome, 25% for genitourinary anomaly, and 12% for others.[35,36]
- End-stage renal disease in patients with WAGR syndrome and genitourinary anomalies tended to occur relatively late, and often during or after adolescence.[35]
Predicting Risk of Kidney Failure
CCSS investigators developed prediction models for kidney failure using demographic and treatment characteristics of 5-year survivors of childhood cancer. The models were validated using follow-up data from survivors in the SJLIFE study and National Wilms Tumor Study.[37]
- In the CCSS, survivor-reported history of subsequent kidney failure (i.e., dialysis, kidney transplant, or kidney-related death) by age 40 years was confirmed by linkage with the Organ Procurement and Transplantation Network and the National Death Index.
- Models integrated clinical data such as race and ethnicity, age at diagnosis, nephrectomy, chemotherapy, radiation therapy, congenital genitourinary anomalies, and early-onset hypertension to estimate the relationships between potential predictors and kidney failure and magnitude of risk.
- The risk prediction models accurately identify survivors at low, moderate, and high risk of late kidney failure who may benefit from screening and intervention.
- The online calculator can be found on the CCSS website.
Therapy-Related Bladder Complications
Pelvic or central nervous system surgery, alkylator-containing chemotherapy such as cyclophosphamide or ifosfamide, pelvic radiation therapy, and certain spinal and genitourinary surgical procedures have been associated with urinary bladder late effects, as follows:
Chemotherapy
- The oxazaphosphorine alkylating agents (cyclophosphamide and ifosfamide) and radiation therapy exposing the bladder have been implicated in the development of hemorrhagic cystitis.
- Chemotherapy-associated hemorrhagic cystitis presents as an acute toxicity and appears to be a rare persistent effect among clinically well characterized long-term survivor cohorts.[38,39]
- In a study of 6,119 children treated between 1986 and 2010 (mean age, 12.2 years ± 6.3 standard deviation), 1.6% of patients (n = 97) developed hemorrhagic cystitis (manifesting at mean 2.7 months after transplant induction therapy and mean 12.4 months after pelvic radiation), most of whom (75%) had severity scores of II or III (scale, I–IV).[40]
- Patients with radiological evidence of renal or bladder calculi or tumors that invaded the bladder wall were excluded from the study.
- Older age, previous bone marrow or peripheral blood HSCT, and BK virus in the urine were risk factors for hemorrhagic cystitis and were associated with a higher severity score.
- Previous exposure to cyclophosphamide has been linked to risk of bladder carcinoma. An excess prevalence of bladder tumors has also been observed in survivors of specific diagnostic types (e.g., heritable retinoblastoma), supporting the contribution of genetic factors in the development of subsequent neoplasms.[41,42]
Radiation therapy
- Pelvic radiation therapy is also associated with an increased risk of hemorrhagic cystitis that may be either acute or chronic in presentation.
- The risk of radiation-induced hemorrhagic cystitis is greatest among survivors treated with radiation doses of more than 30 Gy to the whole bladder or more than 60 Gy to a portion of the bladder.[43]
- Long-term bladder fibrosis and contracture may result as sequelae from hemorrhagic cystitis or radiation therapy.[43]
Surgery
- Surgical procedures involving the lower genitourinary tract have the potential to impair normal function of the bladder and normal voiding mechanisms.
- Any cancer therapy or tumor infiltration that disrupts innervation of the bladder can have deleterious effects on bladder function that may manifest as impaired bladder storage, inability to void, and/or incontinence.
- Children who have undergone ileal enterocystoplasty for bladder augmentation are at risk of developing a vitamin B12 deficiency. Serum B12 levels decrease over time after the procedure, with the greatest risk occurring 7 years postoperatively.[44]
Kidney Transplant
In a study of solid organ transplants in 13,318 survivors in the CCSS, 71 survivors had end-stage kidney disease that warranted kidney transplants, 50 of whom received a kidney transplant.[45]
- At 35 years after cancer diagnosis, the cumulative incidence of a kidney transplant was 0.39%, and the cumulative incidence of being placed on the waiting list or receiving a kidney was 0.54%.
- Exposure to ifosfamide and receiving TBI were associated with the highest hazard ratio for being placed on the waiting list or receiving a kidney transplant.
- The 5-year survival rate from the time of kidney transplant was 93.5%, which is similar to that of the general population in the same age range.
Table 21 summarizes kidney and bladder late effects and the related health screenings.
Table 21. Kidney and Bladder Late Effectsa
Predisposing Therapy |
Renal/Genitourinary Effects |
Health Screening |
BUN = blood urea nitrogen; NSAIDs = nonsteroidal anti-inflammatory drugs; RBC/HFP = red blood cells per high-field power (microscopic examination). |
a Adapted from theChildren's Oncology Group Long-Term Follow-Up Guidelines for Survivors of Childhood, Adolescent, and Young Adult Cancers. |
Cisplatin/carboplatin; ifosfamide; calcineurin inhibitors |
Renal toxicity (glomerular injury, tubular injury [renal tubular acidosis], Fanconi syndrome, hypophosphatemic rickets) |
Blood pressure |
BUN, Creatinine, Na, K, Cl, CO2, Ca, Mg, PO4 levels |
Urinalysis |
Electrolyte supplements for patients with persistent electrolyte wasting |
Nephrology consultation for patients with hypertension, proteinuria, or progressive renal insufficiency |
Methotrexate; radiation exposing kidneys/urinary tract |
Renal toxicity (renal insufficiency, hypertension) |
Blood pressure |
BUN, Creatinine, Na, K, Cl, CO2, Ca, Mg, PO4 levels |
Urinalysis |
Nephrology consultation for patients with hypertension, proteinuria, or progressive renal insufficiency |
Nephrectomy |
Renal toxicity (proteinuria, hyperfiltration, renal insufficiency) |
Blood pressure |
BUN, Creatinine, Na, K, Cl, CO2, Ca, Mg, PO4 levels |
Urinalysis |
Discuss contact sports, bicycle safety (e.g., avoiding handlebar injuries), and proper use of seatbelts (i.e., wearing lap belts around hips, not waist) |
Counsel to use NSAIDs with caution |
Nephrology consultation for patients with hypertension, proteinuria, or progressive renal insufficiency |
Nephrectomy; pelvic surgery; cystectomy |
Hydrocele |
Testicular examination |
Cystectomy |
Cystectomy-related complications (chronic urinary tract infections, renal dysfunction, vesicoureteral reflux, hydronephrosis, reservoir calculi, spontaneous neobladder perforation, vitamin B12 /folate/carotene deficiency [patients with ileal enterocystoplasty only]) |
Urology evaluation |
Vitamin B12 level |
Pelvic surgery; cystectomy |
Urinary incontinence; urinary tract obstruction |
History: hematuria, urinary urgency/frequency, urinary incontinence/retention, dysuria, nocturia, abnormal urinary stream |
Counsel regarding adequate fluid intake, regular voiding, seeking medical attention for symptoms of voiding dysfunction or urinary tract infection, compliance with recommended bladder catheterization regimen |
Urologic consultation for patients with dysfunctional voiding or recurrent urinary tract infections |
Cyclophosphamide/Ifosfamide; radiation exposing bladder/urinary tract |
Bladder toxicity (hemorrhagic cystitis, bladder fibrosis, dysfunctional voiding, vesicoureteral reflux, hydronephrosis) |
History: hematuria, urinary urgency/frequency, urinary incontinence/retention, dysuria, nocturia, abnormal urinary stream |
Urinalysis |
Urine culture, spot urine calcium/creatinine ratio, and ultrasound of kidneys and bladder for patients with microscopic hematuria (defined as ≥5 RBC/HFP on at least 2 occasions) |
Nephrology or urology referral for patients with culture-negative microscopic hematuria AND abnormal ultrasound and/or abnormal calcium/creatinine ratio |
Urology referral for patients with culture negative macroscopic hematuria |
For urinary late effects information, including risk factors, evaluation, and health counseling, see the Children's Oncology Group Long-Term Follow-Up Guidelines for Survivors of Childhood, Adolescent, and Young Adult Cancers.
References:
-
Shnorhavorian M, Friedman DL, Koyle MA: Genitourinary long-term outcomes for childhood cancer survivors. Curr Urol Rep 10 (2): 134-7, 2009.
-
Green DM: Evaluation of renal function after successful treatment for unilateral, non-syndromic Wilms tumor. Pediatr Blood Cancer 60 (12): 1929-35, 2013.
-
Dieffenbach BV, Murphy AJ, Liu Q, et al.: Cumulative burden of late, major surgical intervention in survivors of childhood cancer: a report from the Childhood Cancer Survivor Study (CCSS) cohort. Lancet Oncol 24 (6): 691-700, 2023.
-
Dieffenbach BV, Liu Q, Murphy AJ, et al.: Late-onset kidney failure in survivors of childhood cancer: a report from the Childhood Cancer Survivor Study. Eur J Cancer 155: 216-226, 2021.
-
Kooijmans ECM, van der Pal HJH, Pluijm SMF, et al.: The Dutch Childhood Cancer Survivor Study (DCCSS)-LATER 2 kidney analysis examined long-term glomerular dysfunction in childhood cancer survivors. Kidney Int 102 (5): 1136-1146, 2022.
-
Kooijmans ECM, van der Pal HJH, Pluijm SMF, et al.: Long-Term Tubular Dysfunction in Childhood Cancer Survivors; DCCSS-LATER 2 Renal Study. Cancers (Basel) 14 (11): , 2022.
-
Mansouri I, Schwartz B, Vu-Bezin G, et al.: Risk of Renal or Urinary Related Hospitalization in Survivors of Childhood Cancer: Results from the French Childhood Cancer Survivor Study. Cancer Epidemiol Biomarkers Prev 32 (4): 572-581, 2023.
-
Chao C, Bhatia S, Xu L, et al.: Chronic Comorbidities Among Survivors of Adolescent and Young Adult Cancer. J Clin Oncol 38 (27): 3161-3174, 2020.
-
Dekkers IA, Blijdorp K, Cransberg K, et al.: Long-term nephrotoxicity in adult survivors of childhood cancer. Clin J Am Soc Nephrol 8 (6): 922-9, 2013.
-
Mulder RL, Knijnenburg SL, Geskus RB, et al.: Glomerular function time trends in long-term survivors of childhood cancer: a longitudinal study. Cancer Epidemiol Biomarkers Prev 22 (10): 1736-46, 2013.
-
Knijnenburg SL, Jaspers MW, van der Pal HJ, et al.: Renal dysfunction and elevated blood pressure in long-term childhood cancer survivors. Clin J Am Soc Nephrol 7 (9): 1416-27, 2012.
-
Weil BR, Murphy AJ, Liu Q, et al.: Late Health Outcomes Among Survivors of Wilms Tumor Diagnosed Over Three Decades: A Report From the Childhood Cancer Survivor Study. J Clin Oncol 41 (14): 2638-2650, 2023.
-
Khondker A, Jain A, Groff ML, et al.: Late Kidney Effects of Nephron-Sparing vs Radical Nephrectomy for Wilms Tumor: A Systematic Review and Meta-Analysis. J Urol 207 (3): 513-523, 2022.
-
Skinner R, Kaplan R, Nathan PC: Renal and pulmonary late effects of cancer therapy. Semin Oncol 40 (6): 757-73, 2013.
-
Skinner R, Parry A, Price L, et al.: Persistent nephrotoxicity during 10-year follow-up after cisplatin or carboplatin treatment in childhood: relevance of age and dose as risk factors. Eur J Cancer 45 (18): 3213-9, 2009.
-
Marina NM, Poquette CA, Cain AM, et al.: Comparative renal tubular toxicity of chemotherapy regimens including ifosfamide in patients with newly diagnosed sarcomas. J Pediatr Hematol Oncol 22 (2): 112-8, 2000 Mar-Apr.
-
Hartmann JT, Fels LM, Franzke A, et al.: Comparative study of the acute nephrotoxicity from standard dose cisplatin +/- ifosfamide and high-dose chemotherapy with carboplatin and ifosfamide. Anticancer Res 20 (5C): 3767-73, 2000 Sep-Oct.
-
Skinner R, Cotterill SJ, Stevens MC: Risk factors for nephrotoxicity after ifosfamide treatment in children: a UKCCSG Late Effects Group study. United Kingdom Children's Cancer Study Group. Br J Cancer 82 (10): 1636-45, 2000.
-
Stöhr W, Paulides M, Bielack S, et al.: Ifosfamide-induced nephrotoxicity in 593 sarcoma patients: a report from the Late Effects Surveillance System. Pediatr Blood Cancer 48 (4): 447-52, 2007.
-
Oberlin O, Fawaz O, Rey A, et al.: Long-term evaluation of Ifosfamide-related nephrotoxicity in children. J Clin Oncol 27 (32): 5350-5, 2009.
-
Daetwyler E, Bargetzi M, Otth M, et al.: Late effects of high-dose methotrexate treatment in childhood cancer survivors-a systematic review. BMC Cancer 22 (1): 267, 2022.
-
Cohen EP, Robbins ME: Radiation nephropathy. Semin Nephrol 23 (5): 486-99, 2003.
-
Dawson LA, Kavanagh BD, Paulino AC, et al.: Radiation-associated kidney injury. Int J Radiat Oncol Biol Phys 76 (3 Suppl): S108-15, 2010.
-
Mitus A, Tefft M, Fellers FX: Long-term follow-up of renal functions of 108 children who underwent nephrectomy for malignant disease. Pediatrics 44 (6): 912-21, 1969.
-
Bölling T, Ernst I, Pape H, et al.: Dose-volume analysis of radiation nephropathy in children: preliminary report of the risk consortium. Int J Radiat Oncol Biol Phys 80 (3): 840-4, 2011.
-
Peschel RE, Chen M, Seashore J: The treatment of massive hepatomegaly in stage IV-S neuroblastoma. Int J Radiat Oncol Biol Phys 7 (4): 549-53, 1981.
-
Ritchey ML, Green DM, Thomas PR, et al.: Renal failure in Wilms' tumor patients: a report from the National Wilms' Tumor Study Group. Med Pediatr Oncol 26 (2): 75-80, 1996.
-
Paulino AC, Wilimas J, Marina N, et al.: Local control in synchronous bilateral Wilms tumor. Int J Radiat Oncol Biol Phys 36 (3): 541-8, 1996.
-
Cheng JC, Schultheiss TE, Wong JY: Impact of drug therapy, radiation dose, and dose rate on renal toxicity following bone marrow transplantation. Int J Radiat Oncol Biol Phys 71 (5): 1436-43, 2008.
-
Hoffmeister PA, Hingorani SR, Storer BE, et al.: Hypertension in long-term survivors of pediatric hematopoietic cell transplantation. Biol Blood Marrow Transplant 16 (4): 515-24, 2010.
-
Abboud I, Porcher R, Robin M, et al.: Chronic kidney dysfunction in patients alive without relapse 2 years after allogeneic hematopoietic stem cell transplantation. Biol Blood Marrow Transplant 15 (10): 1251-7, 2009.
-
Ellis MJ, Parikh CR, Inrig JK, et al.: Chronic kidney disease after hematopoietic cell transplantation: a systematic review. Am J Transplant 8 (11): 2378-90, 2008.
-
Poppe MM, Tai A, Li XA, et al.: Kidney Disease in Childhood Cancer Survivors Treated With Radiation Therapy: A PENTEC Comprehensive Review. Int J Radiat Oncol Biol Phys 119 (2): 560-574, 2024.
-
Green DM, Wang M, Krasin MJ, et al.: Long-term renal function after treatment for unilateral, nonsyndromic Wilms tumor. A report from the St. Jude Lifetime Cohort Study. Pediatr Blood Cancer 67 (10): e28271, 2020.
-
Breslow NE, Collins AJ, Ritchey ML, et al.: End stage renal disease in patients with Wilms tumor: results from the National Wilms Tumor Study Group and the United States Renal Data System. J Urol 174 (5): 1972-5, 2005.
-
Hamilton TE, Ritchey ML, Haase GM, et al.: The management of synchronous bilateral Wilms tumor: a report from the National Wilms Tumor Study Group. Ann Surg 253 (5): 1004-10, 2011.
-
Wu NL, Chen Y, Dieffenbach BV, et al.: Development and Validation of a Prediction Model for Kidney Failure in Long-Term Survivors of Childhood Cancer. J Clin Oncol 41 (12): 2258-2268, 2023.
-
Hudson MM, Ness KK, Gurney JG, et al.: Clinical ascertainment of health outcomes among adults treated for childhood cancer. JAMA 309 (22): 2371-81, 2013.
-
Landier W, Armenian SH, Lee J, et al.: Yield of screening for long-term complications using the children's oncology group long-term follow-up guidelines. J Clin Oncol 30 (35): 4401-8, 2012.
-
Riachy E, Krauel L, Rich BS, et al.: Risk factors and predictors of severity score and complications of pediatric hemorrhagic cystitis. J Urol 191 (1): 186-92, 2014.
-
Kersun LS, Wimmer RS, Hoot AC, et al.: Secondary malignant neoplasms of the bladder after cyclophosphamide treatment for childhood acute lymphocytic leukemia. Pediatr Blood Cancer 42 (3): 289-91, 2004.
-
Frobisher C, Gurung PM, Leiper A, et al.: Risk of bladder tumours after childhood cancer: the British Childhood Cancer Survivor Study. BJU Int 106 (7): 1060-9, 2010.
-
Ritchey M, Ferrer F, Shearer P, et al.: Late effects on the urinary bladder in patients treated for cancer in childhood: a report from the Children's Oncology Group. Pediatr Blood Cancer 52 (4): 439-46, 2009.
-
Rosenbaum DH, Cain MP, Kaefer M, et al.: Ileal enterocystoplasty and B12 deficiency in pediatric patients. J Urol 179 (4): 1544-7; discussion 1547-8, 2008.
-
Dietz AC, Seidel K, Leisenring WM, et al.: Solid organ transplantation after treatment for childhood cancer: a retrospective cohort analysis from the Childhood Cancer Survivor Study. Lancet Oncol 20 (10): 1420-1431, 2019.
Latest Updates to This Summary (06 / 24 / 2024)
The PDQ cancer information summaries are reviewed regularly and updated as new information becomes available. This section describes the latest changes made to this summary as of the date above.
Late Effects of the Cardiovascular System
Added text about the results of a Childhood Cancer Survivor Study (CCSS) that evaluated radiation dose–response relationships for cardiac substructures and cardiac outcomes among 25,481 5-year survivors treated between 1970 to 1999 (cited Bates et al. as reference 19).
Added text to state that dyslipidemia is more common and is an independent predictor of cardiovascular disease among survivors, compared with controls. Also added text about the results of a St. Jude Life (SJLIFE) cohort study that investigated specific lipid abnormalities and the risk of atherosclerotic cardiovascular disease in 4,115 childhood cancer survivors (cited Goldberg et al. as reference 21).
Added text about the results of a Pediatric Normal Tissue Effects in the Clinic (PENTEC) study that reported on the risk of late cardiac disease for childhood cancer survivors who were treated with chemotherapy or radiation therapy (cited Bates et al. as reference 26).
Added text about the results of four Children's Oncology Group (COG) Hodgkin lymphoma clinical trials spanning from 2002 to 2022, which showed that the risk of late cardiovascular outcomes may be decreasing (cited Lo et al. as reference 52).
Added Mortality Risk After Major Cardiovascular Events as a new subsection.
Added text to state that physical activity has been shown to be a safe intervention against the high burden of cardiovascular late effects in childhood cancer survivors. Also added text about the results of a randomized controlled trial that investigated the effect of a partially supervised, personalized, 1-year physical activity intervention on cardiovascular health in long-term survivors of childhood cancer (cited Rueegg et al. as reference 100).
Late Effects of the Central Nervous System
Added text about the findings from a PENTEC comprehensive review that evaluated the association between methotrexate exposure and IQ score.
Late Effects of the Digestive System
Added text about the results of an SJLIFE cohort study that investigated the prevalence of prediabetes among survivors of childhood cancer, risk factors for progression to diabetes, and association between prediabetes and late cardiovascular events and chronic kidney disease (cited Dixon et al. as reference 85).
Late Effects of the Endocrine System
Added text about the results of a report that included 156 children and adolescents with medulloblastoma who were treated with surgery, risk-adapted photon craniospinal irradiation, and dose-intensive chemotherapy between 2003 and 2013 and found the incidence of hypothyroidism to be 48.4%.
Late Effects of the Reproductive System
Added text about the results of a St. Jude Children's Research Hospital study that reported the cumulative risk of hypogonadism and infertility in a cohort of 156 pediatric patients with medulloblastomas who were treated with surgery, risk-adapted craniospinal irradiation, and dose-intensive chemotherapy (cited Merchant et al. as reference 4).
Added text about the results of a retrospective longitudinal analysis that investigated pubertal attainment and Leydig cell function in 130 males who were younger than 18 years when they underwent a transplant (cited Cattoni et al. as reference 38).
Added text about the results of a retrospective observational study that investigated ovarian function after HSCT in 178 women survivors of childhood leukemia from the long-term French follow-up program (cited Chabut et al. as reference 60).
Added text about the development and validation of treatment-informed prediction models to estimate survivors' risks of developing primary ovarian insufficiency (cited Im et al. as reference 62).
Added text about the results of a retrospective observational study that investigated spontaneous pregnancy after HSCT in 178 women survivors of childhood leukemia from the long-term French follow-up program.
Added text about the results of a study that reviewed the effects of radiation therapy to the abdomen on pregnancy and pregnancy outcomes in patients with a history of Wilms tumor.
Late Effects of the Special Senses
Added text to state that hearing sensitivity is measured by pure-tone audiometry. This assessment does not measure the social and emotional impact of hearing loss. Also added text about the results of an SJLIFE cohort study that examined the functional impact of treatment-induced hearing loss on quality of life in adult survivors of childhood cancer (cited Bass et al. as reference 33).
This summary is written and maintained by the PDQ Pediatric Treatment Editorial Board, which is editorially independent of NCI. The summary reflects an independent review of the literature and does not represent a policy statement of NCI or NIH. More information about summary policies and the role of the PDQ Editorial Boards in maintaining the PDQ summaries can be found on the About This PDQ Summary and PDQ® Cancer Information for Health Professionals pages.
About This PDQ Summary
Purpose of This Summary
This PDQ cancer information summary for health professionals provides comprehensive, peer-reviewed, evidence-based information about the late effects of treatment for childhood cancer. It is intended as a resource to inform and assist clinicians in the care of their patients. It does not provide formal guidelines or recommendations for making health care decisions.
Reviewers and Updates
This summary is reviewed regularly and updated as necessary by the PDQ Pediatric Treatment Editorial Board, which is editorially independent of the National Cancer Institute (NCI). The summary reflects an independent review of the literature and does not represent a policy statement of NCI or the National Institutes of Health (NIH).
Board members review recently published articles each month to determine whether an article should:
- be discussed at a meeting,
- be cited with text, or
- replace or update an existing article that is already cited.
Changes to the summaries are made through a consensus process in which Board members evaluate the strength of the evidence in the published articles and determine how the article should be included in the summary.
The lead reviewers for Late Effects of Treatment for Childhood Cancer are:
- Sally J. Cohen-Cutler, MD, MS (Children's Hospital of Philadelphia)
- Louis S. Constine, MD (James P. Wilmot Cancer Center at University of Rochester Medical Center)
- Nita Louise Seibel, MD (National Cancer Institute)
- Emily S. Tonorezos, MD, MPH (National Cancer Institute)
Any comments or questions about the summary content should be submitted to Cancer.gov through the NCI website's Email Us. Do not contact the individual Board Members with questions or comments about the summaries. Board members will not respond to individual inquiries.
Levels of Evidence
Some of the reference citations in this summary are accompanied by a level-of-evidence designation. These designations are intended to help readers assess the strength of the evidence supporting the use of specific interventions or approaches. The PDQ Pediatric Treatment Editorial Board uses a formal evidence ranking system in developing its level-of-evidence designations.
Permission to Use This Summary
PDQ is a registered trademark. Although the content of PDQ documents can be used freely as text, it cannot be identified as an NCI PDQ cancer information summary unless it is presented in its entirety and is regularly updated. However, an author would be permitted to write a sentence such as "NCI's PDQ cancer information summary about breast cancer prevention states the risks succinctly: [include excerpt from the summary]."
The preferred citation for this PDQ summary is:
PDQ® Pediatric Treatment Editorial Board. PDQ Late Effects of Treatment for Childhood Cancer. Bethesda, MD: National Cancer Institute. Updated <MM/DD/YYYY>. Available at: https://www.cancer.gov/types/childhood-cancers/late-effects-hp-pdq. Accessed <MM/DD/YYYY>. [PMID: 26389273]
Images in this summary are used with permission of the author(s), artist, and/or publisher for use within the PDQ summaries only. Permission to use images outside the context of PDQ information must be obtained from the owner(s) and cannot be granted by the National Cancer Institute. Information about using the illustrations in this summary, along with many other cancer-related images, is available in Visuals Online, a collection of over 2,000 scientific images.
Disclaimer
Based on the strength of the available evidence, treatment options may be described as either "standard" or "under clinical evaluation." These classifications should not be used as a basis for insurance reimbursement determinations. More information on insurance coverage is available on Cancer.gov on the Managing Cancer Care page.
Contact Us
More information about contacting us or receiving help with the Cancer.gov website can be found on our Contact Us for Help page. Questions can also be submitted to Cancer.gov through the website's Email Us.
Last Revised: 2024-06-24